Multiple Exciton Generation: Harnessing Solar Energy Efficiently
Definition: Multiple exciton generation (MEG) is a phenomenon in which a single photon absorbed by a semiconductor nanocrystal can generate multiple electron-hole pairs, or excitons. This process has the potential to significantly enhance the efficiency of solar energy conversion devices, such as photovoltaic cells, by overcoming the Shockley-Queisser limit.
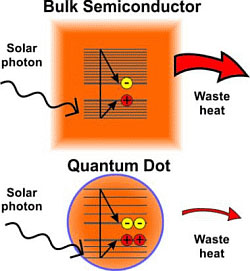
The Physics Behind Multiple Exciton Generation
In a conventional semiconductor, the absorption of a photon with energy greater than the bandgap results in the generation of a single electron-hole pair. Any excess energy is rapidly lost as heat through a process called phonon emission. However, in nanoscale semiconductors, such as quantum dots, the excess energy can be utilized to create additional excitons before phonon emission occurs, leading to MEG.
The efficiency of MEG depends on several factors, including the size and composition of the nanocrystal, the energy of the absorbed photon, and the competition between MEG and other relaxation processes. Quantum confinement in nanocrystals enhances the MEG efficiency by slowing down the phonon emission rate and increasing the Coulomb interaction between the electrons and holes.
Impact on Solar Energy Conversion
MEG has the potential to revolutionize solar energy conversion by significantly increasing the power conversion efficiency of photovoltaic devices. In a conventional solar cell, the maximum theoretical efficiency is limited to about 33% by the Shockley-Queisser limit. This limitation arises because high-energy photons generate hot carriers that quickly thermalize, losing their excess energy as heat.
By harnessing MEG, it is possible to convert a larger fraction of the absorbed solar energy into electrical energy. Theoretically, MEG-based solar cells could achieve power conversion efficiencies exceeding 44% under standard solar illumination conditions. This enhancement in efficiency would lead to a substantial reduction in the cost of solar energy production.
Challenges and Recent Advancements
Despite the promise of MEG, several challenges need to be addressed for its practical implementation in solar energy conversion devices. One of the main challenges is the efficient extraction of the generated multiple excitons before they recombine. The recombination of excitons can occur on a sub-nanosecond timescale, competing with charge extraction processes.
Recent advancements in the field have focused on strategies to enhance MEG efficiency and prolong the lifetime of the generated excitons. These strategies include:
- Tailoring the size, shape, and composition of the nanocrystals to optimize MEG performance
- Developing advanced device architectures that facilitate efficient charge extraction
- Exploring new materials, such as perovskite nanocrystals, that exhibit strong MEG effects
- Investigating the role of surface chemistry and ligands in controlling MEG efficiency
Beyond Solar Energy: Other Applications of MEG
Apart from solar energy conversion, MEG has potential applications in other optoelectronic devices, such as:
- Photodetectors: MEG can enhance the sensitivity and spectral response of photodetectors, enabling the detection of low-intensity and broadband optical signals.
- Light-emitting devices: The reverse process of MEG, known as multi-exciton recombination, can be exploited to generate high-intensity and tunable light emission from nanocrystal-based light-emitting diodes (LEDs).
- Quantum information processing: MEG in nanocrystals can be used to generate entangled photon pairs, which are essential for quantum communication and quantum computing applications.
Conclusion
Multiple exciton generation is a fascinating phenomenon that holds great promise for enhancing the efficiency of solar energy conversion and advancing various optoelectronic applications. By harnessing the unique properties of semiconductor nanocrystals, MEG offers a route to overcome the fundamental limitations of conventional devices.
As research in this field continues to progress, we can expect to see new breakthroughs in materials design, device architecture, and fundamental understanding of the MEG process. The successful implementation of MEG in practical devices will not only revolutionize solar energy production but also open up exciting opportunities in other areas of nanotechnology and optoelectronics.
Further Reading
Nanomaterials, Multiple Exciton Generation in Colloidal Nanocrystals
Journal of Nanotechnology, Multiple Exciton Generation in Nanostructures for Advanced Photovoltaic Cells
Nanophotonics, Multiple exciton generation in quantum dot-based solar cells