Posted: Feb 19, 2016 |
Could the future of low-power computing be magnetism?
(Nanowerk News) Researchers at the U.S. Department of Energy's (DOE's) Argonne National Laboratory have made two recent advances in the field of spin-wave logic, or the potential use of magnetic spins to transmit and manipulate data.
|
Computer hard drives, credit cards and digital video recorders all store data through static magnetic memory, but before that data can be processed or transferred, it must be converted to an electrical current. However, scientists are interested in also using magnetic films to process and transfer data, which could make for faster, lower power devices than today's electronics and computing devices.
|
"In semiconductor electronics, information is encoded and transported in electronic charges through electrons," said Axel Hoffmann, senior materials scientist. "It is conceivable that information could also be transferred as magnetic spins in magnetic materials."
|
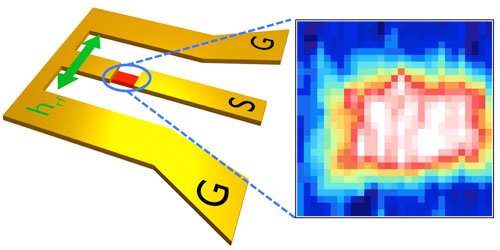 |
On the left, a schematic shows the experimental setup for measuring spin dynamics in a sample of YIG. On the right, a Brillouin light scattering map of a micro-sized bar of YIG excited via an electrical current through a platinum overlayer reveals a strong spin-wave localization in the center of the sample known as a “bullet.” The color red indicates a high-spin wave intensity and the color blue indicates an absence of spin waves. (Image provided by M. Benjamin Jungfleisch)
|
Because the research is still exploratory, scientists cannot be positive how much less power a magnetic computing device would use than an electronic computing device. But at the fundamental level, Hoffmann said, semiconductor electronics can be pushed to run at gigahertz frequencies — one billion cycles per second, which is a measurement of energy in the system — while energized magnets start at gigahertz frequencies and speed up as the system size shrinks.
|
"In today's computers, there is energy associated with charge, so when charges change to convey information, you lose energy," Hoffman said. "But in magnetic systems, information could be conveyed based on two energetically identical ways for magnetic spins to point. This would use significantly less energy. At the same time, a thousandfold increase in speed might be possible."
|
For recent papers published in Nanoscale ("Epitaxial patterning of nanometer-thick Y3Fe5O12 films with low magnetic damping") and Physical Review Letters ("Large Spin-Wave Bullet in a Ferrimagnetic Insulator Driven by the Spin Hall Effect"), Argonne researchers worked with the magnetic insulator yttrium iron garnet (YIG), a compound that has been important to magnetic dynamics research but has rarely been investigated for its potential applications. YIG is a particularly good magnetic system candidate for low-power devices because it has low magnetic damping — essentially, low friction or energy dissipation.
|
Because electronic devices are conducting or semiconducting, the techniques used to fabricate these devices have seldom been applied to insulating materials. In Nanoscale, the Argonne researchers demonstrate a method for fabricating a nanopatterned YIG film BY using techniques already widely used in the electronics industry: sputtering and lithographic patterning. Using resources from Argonne's Center for Nanoscale Materials, a DOE Office of Science User Facility, researchers modified these two techniques to create nanometer-thick films with nanopatterned wires and dots that retain the desired properties of YIG.
|
"Nanowires and nanodots control how the information is propagated," said Wei Zhang, postdoctoral associate and an author of the Nanoscale paper.
|
Like the thin line of a needle on a compass, Hoffmann added, the shape of the nanostructure directs the magnons — the small units of energy that cause atoms in a magnetic material to reverse their spin.
|
Researchers also studied the nonlinear magnetic dynamics of YIG on the larger microscale in Physical Review Letters. They sent an electrical current through a layer of platinum on top of the YIG sample to electrically excite the YIG, then used a technique called Brillouin light scattering (BLS) spectroscopy to image spin dynamics. Under the "spotlight" of BLS spectroscopy, researchers found that YIG did not behave as they expected.
|
"By interrogating magnetic dynamics with light, it allows us to look at how excited magnetic spins are spatially distributed," said M. Benjamin Jungfleisch, postdoctoral associate and lead author.
|
Without adequate information on the nonlinear dynamics of YIG, the research community has had to assume that its dynamics are the same throughout the material.
|
"These materials have always been analyzed as if they were homogeneous, but they're not," Jungfleisch said. "We saw that they are responding heterogeneously even though we excited them the same everywhere."
|
Despite electrically exciting the material uniformly, they found that most of the magnetic energy focused into a concentrated area known as a "bullet." This finding is important to future applications of YIG thin films because it demonstrates unique effects of combining magnetism and electrical current.
|
"All our common technology uses electrical currents," said Hoffmann. "So we have to figure out how to connect one world with another if we're to find workable applications for spin-wave logic."
|
The researchers' next step is to use BLS spectroscopy on nanopatterned YIG thin films to observe magnetic dynamics as they would occur on the scale required for power applications.
|