Posted: Apr 29, 2016 |
Magnetic nanoparticles may reveal early traces of cancer
(Nanowerk News) Nanoscale magnets offer a new way to find faint, early traces of cancer in patients, according to Rice University students working on a method to capitalize on the magnets’ properties. Three Rice computational and applied mathematics students are refining a program to analyze magnetic relaxometry signals from iron-oxide nanoparticles that find and attach themselves to cancerous cells.
|
Rice seniors Brian Ho, Rachel Hoffman and Eric Sung have developed a novel way to analyze data for cancer researchers who hope to use magnetic nanoparticles to locate signs of cancer that X-rays would never spot.
|
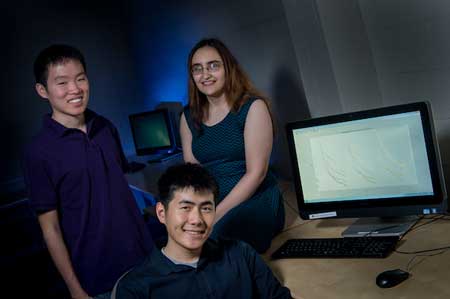 |
Rice engineering students are helping to maximize the promise of magnetic nanoparticles to find faint traces of cancer in patients. From left: Brian Ho, Eric Sung and Rachel Hoffman.
|
All magnets (or materials prone to magnetism) have magnetic “moments,” like invisible needles that can move and react to magnetic fields, even if their physical hosts can’t.
|
These ghostly needles align when exposed to an external magnetic field; when the field is removed, they “relax” once again. Relaxometry measures this latter characteristic. It turns out the moments relax at a very different rate when they belong to nanoparticles that are bound to cancer cells.
|
The students are working with Rice adviser Béatrice Rivière, the Noah G. Harding Chair and a professor of computational and applied mathematics, and doctors at the University of Texas MD Anderson Cancer Center in Houston to develop computer programs that analyze “traces” of these moments as they relax. Albuquerque, N.M.,-based Senior Scientific, in collaboration with MD Anderson, is developing a commercial relaxometry platform for the early detection of cancer.
|
The 25-nanometer superparamagnetic iron-oxide nanoparticles are enhanced with antibody proteins that target biomarker proteins produced by cancer cells, Sung said. “Once they bind to the cells, their range of motion is severely restricted, and this restricted movement is pretty important,” he said. “Once you apply an external magnetic field, the particles’ dipoles will align to counteract the field. Once the dipoles face each other, then you have a magnetic field of essentially zero. But the interesting part to us is what comes after.”
|
The students and the MD Anderson team are working to quantify this relaxation phase because it marks the location of cancer cells in lab samples and in mice.
|
Unbound nanoparticles will randomly reorient themselves in less than a millisecond, but because antibody-associated nanoparticle complexes that are bound to cancer cells are restricted in their movement, their magnetic relaxation is a lot slower – up to a second, Sung said. “We’re figuring out exactly what that means.” he said.
|
The team noted today’s best cancer detection methods only catch tumors with more than 10 million cancer cells. The new approach has the potential to detect tumors with as few as 20,000 cells. The students expect methods that rely on relaxometry will also be safer than current methods that expose patients to ionizing radiation.
|
The students’ software addresses two problems that can corrupt relaxometry data. One is that physical motion – like a patient’s breathing – can displace the target signal and skew the results. The other is what the students call “flux jumps,” a recording artifact that causes a wholesale shift in the data. “The flux jump has to do with the way it’s measured,” Sung said. “But we’ve figured out an algorithm to take care of both these things. And it looks pretty nice.”
|
Hoffman said the Rice team brought new perspective to the problem recognized by MD Anderson’s David Fuentes, an assistant professor in the Department of Imaging Physics, and his colleagues. “They were looking at it very theoretically, whereas we look at it more pragmatically,” she said. “We researched what we can do with this particular data, as opposed to trying to develop an algorithm that could be applied to any data set.”
|
“Indeed, the senior design team’s contribution to motion correction and flux-jump detection will have a lasting impact and will be incorporated into future analysis pipelines,” Fuentes said.
|
Ho said the Rice team’s next step is to create a way to generate synthetic data traces to test the program. “Once we’re able to put in some flux jumps and breathing spikes, we can quantify how good our algorithm is,” he said.
|