Posted: Aug 08, 2017 |
Development of molecular container with caps that can regulate uptake/release of objects
(Nanowerk News) Crown ethers, first synthesized by Dr. Charles Pedersen (Du Pont, USA) in 1962, were the first artificial macrocyclic host molecules*1. They can capture metal ions in their cavity through coordination with their multiple oxygen atoms (Figure 1a).
|
Owing to the importance of crown ethers, Dr. Pedersen received the Nobel Prize in Chemistry together with two other eminent scientists in 1987. Chemicals similar to crown ethers have been synthesized, and are of great interest for capturing toxins from the environment and chemical sensors. Since host molecules can capture a guest molecule or ion in their cavity, these molecules could be regarded as artificial containers of miniscule volume.
|
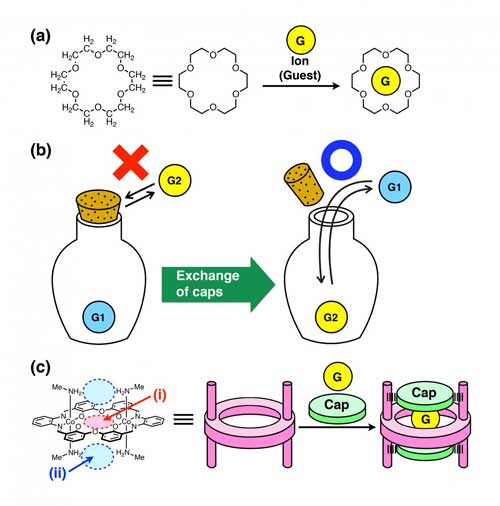 |
(a) Structure of a crown ether (18-crown-6), which can capture a metal ion in the cavity. (b) A bottle with a cork. We cannot put in and take out an object inside the bottle when tightly closed, but we can do so when a loose stopper is used. (c) Metallohost with caps. (i) The host can capture a guest cation in the central crown-ether-like cavity, and (ii) the caps (anions) can be introduced above and below the cavity. (Image: Kanazawa University)
|
A host molecule in general can capture an ion in less than 1 millisecond, so it is indeed an instantaneous reaction. Because most ions can freely enter and exit from the cavity, host molecules so far created can be regarded as molecular containers without a cap (lid).
|
In contrast, an ordinary container used in our daily life comes with a cap (lid), which allows us to keep objects inside or to get them in and out freely (Figure 1b). This function may seem natural, but it was considered to be difficult to confer this kind of function to molecular containers.
|
In order to develop molecular containers with cap functions for more practical applications like storage and transport of molecules or uptake/release of target molecules at will, it is necessary to develop technologies to control such cap-opening and closing just like 'open sesame!'
|
In other words, it is desired to develop mechanisms to make uptake/release of target molecules controllable on our everyday time scale - seconds, minutes or hours.
|
In the present study (Nature Communications, "Anion-capped metallohost allows extremely slow guest uptake and on-demand acceleration of guest exchange"), the research team of Kanazawa University designed and synthesized a novel macrocyclic host molecule, a metallohost, structurally similar to a crown ether, but with caps.
|
The team aimed at developing a new host-guest system by using this novel macrocyclic molecule with exchangeable caps, which allows the speed of guest ion's entering and exiting to be freely adjustable.
|
The research team has successfully obtained the following results.
|
1. Development of a macrocyclic host molecule with caps
|
Based on crown ether, a novel macrocyclic metallohost molecule was synthesized having four CH3NH2 groups, two at the top side and two at the bottom side of the molecule (Figure 1c). This novel macrocyclic host molecule can capture a metal ion of a wide variety (Na+, K+, Rb+, Cs+, Ca2+, La3+). Triflate was introduced at the capping positions; one triflate was located at the top and another at the bottom of the cavity. X-ray crystal structure analysis revealed that triflates are held in place by hydrogen bonds*2 with the CH3NH2 groups.
|
2. Regulation of ion uptake/release by exchanging of the caps
|
The process of ion uptake/release by a macrocyclic host molecule such as a crown ether is generally extremely fast, usually completed in less than 1 millisecond. On the other hand, the novel macrocyclic host molecule developed in this study has been found to capture ions very slowly when the caps are introduced.
|
In particular, La3+ was captured extremely slowly; it took more than 120 hours for its completion. It is thought that triflate caps cover the cavity, preventing La3+ from accessing the binding space. The rate (speed) of ion uptake depended on the species of the cap to a large extent. When acetate ion was employed as the cap, uptake of La3+ was completed within 5 min; the rate was estimated to be at least 100 times faster than in the case where triflate is used as the cap (Figure 2).
|
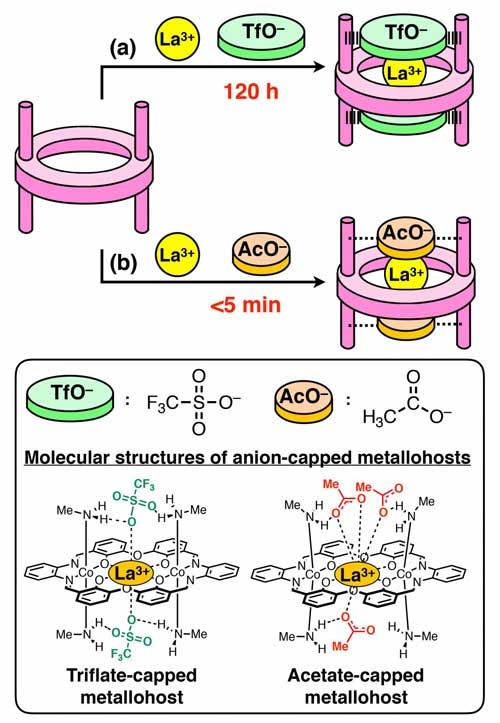 |
Uptake rate of La3+ depends on anions (caps). (a) Triflate, very slow; (b) Acetate, fast. (Image: Kanazawa University)
|
As seen above, the rate of ion uptake by the novel macrocyclic host molecule depends on the cap species. Moreover, triflate caps can easily be exchanged with other cap species since the triflate caps are only held by the macrocyclic host molecule through hydrogen bonds with the CH3NH2 groups.
|
3. Control of the start of ion uptake/release in an 'on-demand' manner
|
Even in a mixture of ions, it is possible to control the start of uptake/release of the target ion by exchanging the caps in an on-demand and time-programmable manner.
|
Herein, + and La3+ were examined; K+ was captured very quickly while La3+ capture was very slow. When triflate was used as the cap, it was found that only K+ was captured even if these two ions were simultaneously mixed with the macrocyclic host molecule (inclusion complex A in Figure 3).
|
 |
(a) Host-guest system in which guest exchange is initiated by exchange of anion caps. (b) Time course of the guest exchange efficiency. (i) When TfO- is used as a counteranion, the guest exchange is very slow. (ii) & (iii) Addition of acetate ion (AcO-) significantly accelerates the guest exchange. (Image: Kanazawa University)
|
Although other analyses indicated that the inclusion complex with La3+ is in fact more stable than that with K+ (metastable state), no conversion to the complex with La3+ was found to take place (inclusion complex B in Figure 3).
|
Indeed, even after two weeks, very little inclusion complex with La3+ was detected. This result indicates that triflate caps nearly completely prevent the ion from entering and exiting. On the other hand, the same experiment with acetate caps in place of triflate caps showed rapid ion exchange, 75 times faster than in the case with triflate caps (Figure 3).
|
Furthermore, when acetate was added 120 hours after the formation of the inclusion complex A, rapid ion exchange started immediately (inclusion complex C in Figure 3).
|
In other words, the cap species can function as an ion-selective regulator for the macrocyclic host, generating a metastable state; this metastable state enables us to control the flow of ions into the host in an on-demand and time-programmable manner by exchanging the caps.
|
So far, many studies have reported on the control of ion uptake/release by host molecules, but most of them relied on external stimuli to change the binding affinity of the host molecules. The present study presents an alternative approach, where the binding affinity is not changed, but rather the formation rate is controlled by caps.
|
A superb example of control of ion uptake/release from the metastable state is the function of ion channels*3 of biological membranes to regulate the intracellular and extracellular ion concentration; this function is indispensable for the survival of living organisms. The novel macrocyclic host molecule has realized a similar function as a single molecule.
|
Future prospect
|
This study has developed a novel method to link the rate and the function of a molecular host through time-programmable control of guest binding. The technology developed herein will enable us to create new host molecules that can control the capture of ions at a given time.
|
The outcome of the present study is expected to open the way for development of precisely time-controllable molecular functions, such as releasing drugs or functional molecules at desired places in a time-programmable manner, driving molecular machines*4 at will.
|
Glossary
|
*1 Host molecule
|
An enzyme binds specific substrate (molecule), like a key and its keyhole, and catalyzes it in a specific manner. A molecule, like an enzyme, that can selectively bind specific molecule or ion, is called the host, while the molecule or ion to be captured, the guest. The research field on host molecules and host-guest interactions is referred to as the host-guest chemistry (molecular recognition chemistry). Cyclodextrin is a natural example of host molecules, while artificially synthesized ones include crown ether, calixarene, pillararene, and so on.
|
*2 Hydrogen bond
|
A hydrogen bond is the electrostatic attraction, non-covalent bond, between two polar groups that occurs when a hydrogen (H) atom covalently bound to a highly electronegative atom such as nitrogen (N), or oxygen (O) experiences the electrostatic field of another highly electronegative atom such as N, O, or fluorine (F) nearby. A hydrogen bond free energy is 5 to 40 kJ/mol, bigger than that of van der Waals force (~1 kJ/mol) but much less than that of a covalent bond (~500 kJ/mol). At a room temperature, a hydrogen bond can be formed and broken in a reversible manner. Therefore, the cap bound to host macrocyclic molecule by hydrogen bonds in the present study could be exchanged with other kinds of caps rather easily.
|
*3 Ion channel
|
A group of transmembrane proteins, having a pore (channel) that allows ions to pass through in a passive manner. An ion can pass through the narrow pore but most ion channels are equipped with a structure called the gate that opens and closes. When and only when the gate is open, an ion can move from one side of the biological membrane to the other side. Ion channels allow ion to move from the side where the ion density is high to the other, where it is low. In contract, an ion pump is a protein that transports ion against the density gradient by utilizing free energy released by ATP hydrolysis.
|
*4 Molecular machine
|
Molecules and/or molecular complexes of a nanometer scale that operate in a controllable manner. Their structure may be changed by external stimuli like light, heat, acid/base, oxidoreduction, and so on. Examples are; molecular motors such as rotaxane and catenane that possess specific structures, like meshing gears, and molecular shuttles. The Nobel Prize in Chemistry 2016 was awarded to Prof. Jean-Pierre Sauvage, Sir James Fraser Stoddart and Prof. Bernard Lucas "Ben" Feringa 'for the design and synthesis of molecular machines.'
|