Posted: Nov 20, 2017 |
Chilled atoms enable deeper understanding of simple chemistry
(Nanowerk News) The field of chemistry often conjures up images of boiling liquids and explosions. But underneath all that eye-catching action is an invisible quantum world where atoms and molecules are constantly rearranging, colliding, and combining to form different molecules.
|
This part of chemistry is rarely seen, but even when scientists do pull back the curtain and expose quantum behavior, the task of understanding chemical reactions at their most fundamental level remains daunting. There are simply too many properties to keep track of for the countless atoms and molecules involved in a reaction. In fact, scientists struggle to keep track of everything even for small chemical reactions, when only a few atoms react.
|
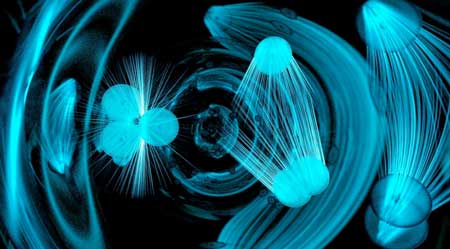 |
Artistic depiction of cold atomic collisions that form molecules. (Image: E. Edwards/JQI)
|
Now, in an article published in the journal Science ("State-to-state chemistry for three-body recombination in an ultracold rubidium gas"), physicists begin to tackle this issue by starting with one of the simplest chemical processes: the formation of a molecule from the collision of three atoms.
|
For the first time, researchers identified all of the quantum attributes of the molecular product while also exerting fine control over how the reaction begins. To achieve this, experimental researchers from the University of Ulm used super-cooled atoms, a platform that allows for exquisite control and observation of many quantum features. In tandem, theorists from the Joint Quantum Institute and JILA developed a mathematical model to simulate the collisions and a University of Hannover physicist provided additional interpretation of the molecular signals.
|
Author Paul Julienne, who is a NIST theorist and JQI Fellow, explains the significance of starting with small reactions. "Calculations involving three objects are hard—Isaac Newton couldn’t do these,” he says. “But we can do some of this rather easily nowadays. Unfortunately, it is almost impossible to do the full quantum dynamics on a computer. Because of this difficulty, these experiments are important for validating the theory so that we can understand this process and perhaps begin to unravel the physics behind larger reactions involving more complex collisions."
|
The experiment begins with a super-cooled atom cloud, trapped within a vacuum environment that is more like space than a chemistry beaker. The atoms have quantum properties, which researchers set precisely using lasers. Occasionally, three atoms get close enough to react and two of them combine into a molecule. The third atom flies away, carrying extra energy from the reaction.
|
Once formed, the molecules become difficult to study because they fall out of the trap. Molecules also have more complex quantum features, such as vibration and rotation, which makes measurement challenging. In order to get over these hurdles, the team uses lasers to strain the molecules according to their quantum properties. Following filtration, they are immediately transferred to a second trap, so that they can be counted. The method is so sensitive it can even detect a single molecule having a specific quantum property.
|
By controlling the starting point of the reaction and implementing this quantum strainer, the team could watch the reaction unfold. They observed that certain quantum properties were mostly constant during molecular formation, while others drastically changed. They were surprised that these cold, slow atoms had enough energy to create molecules with significant rotational energy. And while they initially expected that the molecules would be weakly held together, they found that many were actually tightly bound.
|
While these chemical reactions are far simpler than the sizable molecules that many engineers and industry researchers may want to simulate, they offer insight into the most basic molecular formation. This bottom-up approach may offer guidance to scientists as they model chemical reactions that are impossible to calculate exactly using modern computation.
|
Johannes Hecker Denschlag, one of the experimental authors and a professor at the University of Ulm explains one ultimate goal of this research. "A lot more needs to be investigated, but once we really understand the chemical processes in depth we can use this approach to, for example, control chemical reactions on a new level with quantum tools," he says.
|