Posted: Jan 10, 2018 |
A rare quantum state realized in a new material
(Nanowerk News) An exotic quantum state called a Dirac semimetal was realized in a novel magnetic material Sr1-yMn1-zSb2 (Nature Materials, "A magnetic topological semimetal Sr1-yMn1-zSb2 (y, z < 0.1)"). This material features an unusual combination of two distinct electronic behaviors.
|
One behavior is the persistence of a state similar to a bar magnet with spins that are parallel to each other. The other relates to electrons with an effective mass near zero that travel close to the speed of light.
|
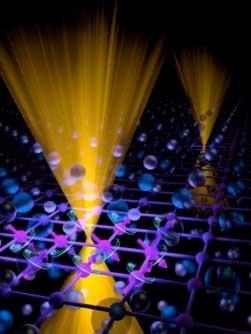 |
The artwork depicts the magnetic and electronic states of the newly discovered magnetic topological semimetal (Sr1-yMn1-zSb2); its unusual and robust electronic behavior enables the flow of electrical current with little resistance. The violet spheres are strontium (Sr), blue is antimony (Sb), and purple is manganese (Mn). Arrows represent the alignment of magnetic moments. The two gold cones touching at a point (called Dirac point) represent the energy and momentum states of conducting electrons. (Image: Oak Ridge National Laboratory)
|
The Dirac semimetal belongs to a class of materials called topological semimetals. These materials offer great promise. They could be the basis for ultra-powerful computers. Essentially, the semimetal could create a new paradigm for highly efficient and robust computational platforms. Semimetals could let such computers and other quantum devices work in warmer settings, climbing further from absolute zero.
|
A 3-D analog of graphene (a 2-D material) is a Dirac semimetal. Dirac semimetals have exceedingly high charge carrier mobility, creating little to no resistance to current flow, and large magnetoresistance that could be used for high-density, low-power data storage.
|
A team led by Tulane University, Louisiana Consortium for Neutron Scattering at Louisiana State University, and others discovered a new magnetic semimetal Sr1-yMn1-zSb2 (y, z<0.1) with nearly massless quantum particles (electrons in this case) that follow the rules of relativistic quantum mechanics.
|
Neutron scattering experiments revealed an unusual combination of ferromagnetic and antiferromagnetic states in the material that vary as a function of temperature. In general, materials exhibit either ferromagnetism or antiferromagnetism, not both in the same material at a given condition.
|
The observed persistence of a ferromagnetic component in the antiferromagnetic state at low temperatures is the signature for the breaking of the time-reversal symmetry (that is, running backward does not return to its starting conditions).
|
The combination of unusual electronic behaviors in this new material offers a rare opportunity to investigate the interplay between relativistic quantum states and spontaneous breaking of symmetry associated with time. These materials offer great promise for highly efficient quantum devices and quantum computers.
|