Feb 08, 2011 |
Keck Foundation funds work on tiny, implantable computers to restore lost brain functions
|
(Nanowerk News) Tiny, implantable computers that would restore brain function lost to disease or injury is the goal of University of Washington research recently funded by a $1 million, three-year grant from the W.M. Keck Foundation.
|
The UW has made significant progress in neural engineering – the study of communication and control between biological and machine systems. The Keck project is the next step in advancing the technology of miniature devices developed at the UW to record from and stimulate the brain, spinal cord and muscles.
|
The principal investigator on the Keck Foundation grant is Dr. Eberhard E. Fetz, UW professor of physiology and biophysics and a core staff researcher at the Washington National Primate Research Center. He and his colleagues have successfully deployed tiny, battery-powered implantable brain-computer interfaces called neurochips in animals.
|
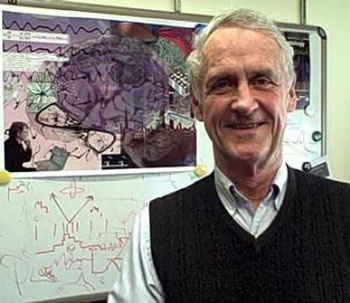 |
Dr. Eberhard Fetz at the University of Washington in Seattle is principal investigator on a W.M. Keck Foundation grant to develop tiny, implantable computers to restore brain functions lost to injury or disease.
|
The neurochip can record nerve cell activity in one part of the brain, process this activity and then stimulate cells in another brain region. The battery-powered device operates continuously during free behavior. When primates carry out their usual daily activities – socializing, climbing, eating, and exploring – their brains can learn to exploit these new resources under normal behavioral conditions.
|
One potential clinical application is to bridge lost biological connections. For example, the researchers have shown that monkeys can learn to bypass an anesthetic block in the nerves of the arm and to activate temporarily paralyzed muscles with activity of cortical neurons. In some ways the device acts as a volition processor, tapping into signals representing the will to move and using them to stimulate the paralyzed muscles to reach targets.
|
"Using an implantable computer interface to implement novel interactions between brain sites opens many fundamentally new research directions," Fetz said, "depending on the site of recording and stimulation, and how these signals are processed and transformed."
|
He explained that a second application is to promote neural plasticity, which could strengthen connections and allow some of the brain's functions to be rescued when impaired. This happens naturally when people recover the ability to move or speak again after a stroke or brain injury. The bidirectional brain computer interface could facilitate this recovery and exploit the brain's innate talent for re-organizing itself as it heals.
|
"We expect that the recurrent type of brain computer interface we are trying to develop," he added, "will eventually have numerous clinical applications for bridging damaged biological pathways and strengthening weak neural connections." For example, signals from the motor-control regions of the brain can be used to stimulate parts of the spinal cord to evoke coordinated movements. This would create connections that could replace lost pathways between the brain and spinal cord, a loss that occurs with strokes and spinal cord injuries.
|
Many labs around the world are working on brain-computer interfaces that convert neural activity to control of external devices such as prosthetic limbs or computer cursors. What makes the recently funded project unusual is that its scientists are developing a recurrent implantable device that would interact bidirectionally with the brain. By operating autonomously and continuously, without the need for connection to external instrumentation, it would facilitate long-term behavioral adaptation and plasticity.
|
The proposed research plans to develop this new paradigm to promote restoration of brain, spine, and muscle function. The work could eventually lead to miniaturized electrical and biological interfaces that operate around the clock on a small amount of power while the wearer goes about his or her usual activities, according to Fetz. He added that, if successful, this implantable technology would advance the ability of subjects to effectively control a brain computer interface by allowing long-term adaptation to consistent contingencies, and would open opportunities for the brain to exploit bidirectional interactions with miniature computers. This implementation of continuous reciprocal interaction goes beyond the existing paradigm of using brain signals to control external devices through tethered connections.
|
As part of the project the team also plans to create a powerful multichannel "Keck Active Electrode Array" with integrated electronics to record and stimulate large numbers of brain sites. This array would operate with electrodes on the surface of the brain and be less invasive than penetrating intracortical electrodes.
|
To overcome the many technical problems in creating safe, effective devices of this nature and realizing their clinical potential, the project depends on a team of UW experts in different fields.
|
Dr. Brian Otis, UW assistant professor of electrical engineering, has extensive experience in wireless sensors and in designing extremely small radios that can be incorporated into other devices. He is also an expert in bioelectronics and the processing of signals with minimal power. His group will design and miniaturize the low power circuitry for the computer and the signal amplifiers, and will work toward harvesting energy to operate the device, perhaps from the body's own heat or muscle activity.
|
Dr. Babak Parviz, the UW McMorrow Innovation Associate Professor of Electrical Engineering, has skill in the fabrication of micro- and nano-scale tools, self-assembled biocompatible machinery, and sensors for detecting very faint signals. His group will create the specialized electrode arrays for recording and stimulation, and will help integrate the miniature electronic systems used in the project.
|
Dr. Jeffrey Ojemann, UW professor of neurological surgery, has expanded his father's original studies on mapping of the human brain to identify critical areas for movement, language, memory and other functions prior to epilepsy surgery. He will bring his extensive knowledge of functional brain mapping and clinical recording of signals from the human brain to the project. He will help design and test the custom computer-enabled electrode arrays for potential applications to patient care.
|
Among the engineering and health issues the team will be addressing are integrating the electronics with the electrode array and making it small enough, finding a reliable source of the low power necessary to operate the system, evaluating any hazards the device might pose or serious long-term side effects, and developing biomaterials that won't cause irritation or be rejected, as well as meeting other safety, performance, and acceptability criteria.
|
"We are extremely grateful to the Keck Foundation for supporting this highly ambitious endeavor," Fetz said. "Looking ahead, we can anticipate that future innovations in nanotechnology, computers and brain science will advance this effort beyond the current state of the art. The grant allows us to be poised to incorporate these advances into the development of more powerful recurrent brain computer interfaces. We expect that these devices will have numerous applications in basic neuroscience research and as well as in clinical care."
|