Jul 07, 2011 |
A chemical detour to quantum criticality
|
(Nanowerk News) Physicists and chemists use different techniques to study essentially the same thing — the nature and behavior of matter. Usually the particular path is of little consequence, because they all lead ultimately to the same truths at the end of the experimental journey. Sometimes, however, choosing one path over another can offer unique and interesting insights along the way, such as when a traveler takes the scenic route instead of a more direct path. A good example is in the study of superconductivity, which is generally the realm of solid-state physicists. But some Princeton University chemists recently teamed with physicists at Argonne National Laboratory to uncover a new insight about a common intermetallic superconducting-type crystal structure.
|
Working at the X-ray Science Division (XSD) high-resolution powder diffraction beamline 11-BM-B at the U.S. Department of Energy Office of Science's Advanced Photon Source at Argonne, the researchers found that by manipulating the parameters of a particular chemical bond within the ThCr2Si2 structure, specifically by breaking a Ge-Ge dimer embedded within SrCo2(Ge1-xPx)2, they could induce the absolute-zero phase transition known as a ferromagnetic quantum critical point (QCP).
|
Unlike the everyday phase transitions with which we're all familiar, such as ice melting into liquid or water boiling into steam, a quantum critical point is a type of phase transition driven not by temperature but by the quantum fluctuations that dominate at absolute zero. Depending on the combination of elements and their structure in a compound, inducing a QCP can produce interesting and useful phenomena such as ferromagnetism and superconductivity. Usually the transition is driven by electron doping or manipulating a physical parameter such as pressure, but the research team found a different way.
|
 |
View inside the 11-BM-B beamline experiment station at Sector 11 of the APS. Lynn Ribaud (left) and Matthew Suchomel (both XSD). The custom multi-crystal detector assembly (top right) delivers the highest resolution powder diffraction data available in the Americas.
|
The paper based on their work, published in Nature Physics ("Ferromagnetic quantum critical point induced by dimer-breaking in SrCo2(Ge1-xPx)2"), was inspired by the work of Nobel Prize-winning chemist Roald Hoffmann, who made some interesting observations in the 1980s about ThCr2Si2-type structures.
|
Hoffmann noted that "in some unexpected way, there's a small embedded molecule inside some subset of these compounds," said Robert Cava of Princeton University, a co-author on the paper. "It was not understood why certain crystal structures were the way they were. To explain some structural problems in this family of intermetallic compounds, Hoffmann came up with the idea that instead of thinking about it like a typical metallic compound, you have to consider the chemical bonding to understand what the electronic state of the system actually is."
|
As a solid-state chemist working on superconductors, Cava realized that the ThCr2Si2 structure, with its embedded molecule, "was one example for which the chemical bonding in this molecule might have an impact on the physical properties of superconductors."
|
The team prepared several series of compounds to determine the impact of the embedded molecule on structural properties. They discovered that "something really dramatic" happened in the SrCo2(Ge1-xPx)2 structure when the Ge-Ge dimer in the embedded phosphorus-germanium molecule is broken by changing composition across the series.
|
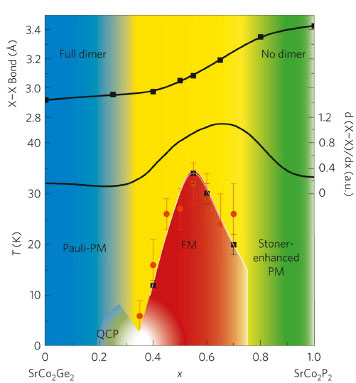 |
From Jia et al. The structural and magnetic phase diagram for SrCo2(Ge1-xPx)2. Black symbols: TC determined by the Arrott plot; the error bars are the temperature interval of the measured isotherms. Red symbols: TC determined by assuming M(TC)=20±10% M (2K). (© 2011 Macmillan Publishers Limited. All rights reserved)
|
"This crazy chemical parameter that we were manipulating somehow induced ferromagnetism and turned it on at near zero Kelvin," said Cava. "When you're approaching a quantum critical point from above in temperature, there are certain characteristics of susceptibility and specific heat that tell you that you're approaching this phase transition at very low temperature. It's in that temperature dependence right around this quantum critical composition that you see the ferromagnetism coming, even though it's not there yet." Another surprising aspect is that such phenomena are typically seen in rare-earth compounds, rather than the transition element compounds used in these experiments.
|
The team's next step will be to grow single crystals of compounds around the QCP in order to study in greater detail the thermodynamics of the approach to the QCP as temperature decreases. Although their work did not result in the discovery of a new path to superconductivity, it offers a new perspective on the structural possibilities in this family of superconducting compounds. "We were asking a fundamental chemical question and found a fundamental physical property. That was just a big surprise," Cava noted. "The idea that this kind of molecule can exist in this structure type impacts the way you think about the iron-arsenide superconductors, which are the ones that could have some kind of application in the future."
|