Feb 26, 2012 |
Graphyne may be better than graphene
|
(Nanowerk News) Super-strong, highly conducting graphene is the hottest ticket in physics, but new computer simulations suggest that materials called graphynes could be just as impressive. Graphynes are one-atom-thick sheets of carbon that resemble graphene, except in the type of atomic bonds. Only small pieces of graphyne have so far been fabricated, but the new simulations, described in Physical Review Letters ("Competition for Graphene: Graphynes with Direction-Dependent Dirac Cones"), may inspire fresh efforts to construct larger samples. The authors show that three different graphynes have a graphenelike electronic structure, which results in effectively massless electrons. The unique symmetry in one of these graphynes may potentially lead to new uses in electronic devices, beyond those of graphene.
|
The singe-atom-thick structure of carbon atoms arranged in a honeycomb pattern, known as graphene, was first isolated in a lab 2004, but many of its remarkable electronic properties were revealed by theorists 60 years before. The most striking aspect of graphene is that its electronic energy levels, or "bands," produce conduction electrons whose energies are directly proportional to their momentum. This is the energy-momentum relationship exhibited by photons, which are massless particles of light. Electrons and other particles of matter normally have energies that depend on the square of their momentum.
|
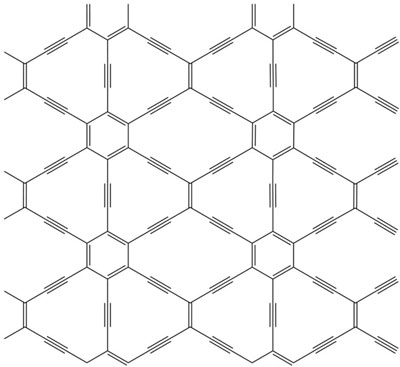 |
Stretched honeycomb. The carbon lattice in this 6,6,12-graphyne has a rectangular symmetry, unlike the hexagonal symmetry of graphene.
|
When the bands are plotted in three dimensions, the photonlike energy-momentum relationship appears as an inverted cone, called a Dirac cone. This unusual relationship causes conduction electrons to behave as though they were massless, like photons, so that all of them travel at roughly the same speed (about 0.3 percent of the speed of light). This uniformity leads to a conductivity greater than copper.
|
Graphynes differ from their carbon cousin graphene in that their 2D framework contains triple bonds in addition to double bonds. These triple bonds open up a potentially limitless array of different geometries beyond the perfect hexagonal lattice of graphene, although only small pieces of graphynes have been synthesized so far. Still, this hasn't stopped theorists from exploring their properties ("Structure-property predictions for new planar forms of carbon: Layered phases containing sp2 and sp atoms"). Recent work gave an indication that certain graphynes might have Dirac cones ("Graphene allotropes"). To verify this, Andreas Görling of the University of Erlangen-Nürnberg in Germany and his colleagues have now performed a more rigorous investigation of graphyne using state-of-the-art methods.
|
The team selected three graphynes to study: two with hexagonal symmetry and a third with rectangular symmetry. The researchers first checked that these graphynes were stable by simulating their vibrations and checking that they returned to their original shape. They then determined the band structure using density-functional theory, the gold standard for dealing with the hopelessly large number of electron-electron interactions inside a material. The simulations showed that all three graphynes had Dirac cones. This was surprising in the case of the rectangular graphyne, Görling says, because most people assumed this sort of electronic structure was tied to hexagonal symmetry. The implication is that many other materials (some containing atoms other than carbon) could have Dirac cones.
|
On closer examination of the rectangularly symmetric graphyne, the team discovered that the Dirac cones were not perfectly conical. A vertical slice in the direction of the "short side" of the rectangular lattice gave an inverted triangle as would be expected, but in the perpendicular direction, parallel to the "long side," the cross section was curved, like a triangle bent towards a parabola. This distortion should lead to a conductance that depends on the direction of the current, a property not found in graphene but one that could be exploited in nanoscale electronic devices, Görling says. Another potentially useful property of this graphyne is that it should naturally contain conducting electrons and should not require noncarbon "dopant" atoms to be added as a source of electrons, as is required for graphene.
|
The big challenge now is to make large graphyne samples. "Organic chemists like myself can synthesize (often with difficulty) complex molecular subunits," but these small sections of graphyne do not exhibit the expected properties of a large lattice, says Michael Haley of the University of Oregon in Eugene. Andre Geim of the University of Manchester, UK, who was awarded the 2010 Nobel Prize for his experimental work on graphene, says that graphyne is "an extremely interesting material, and this report adds to the excitement." He only hopes it won't take 60 years for experimentalists to make the excitement a reality this time.
|