Jan 22, 2015 |
From stem cell to nerve cell in a few weeks
|
(Nanowerk News) Many clinicians have pinned their hopes on stem cells. Stem cells could, for example, replace nerve cells after a stroke. However, until now it has not been possible to observe the process of live stem cells developing into fully functional nerve cells. Now, researchers at the Max Planck Institute for Metabolism Research in Cologne have developed a novel method for studying such processes in the living brain, enabling them to observe how human stem cells transplanted into the cerebral cortex of mice develop into mature functional nerve cells (Biomaterials, "Human neural stem cell intracerebral grafts show spontaneous early neuronal differentiation after several weeks").
|
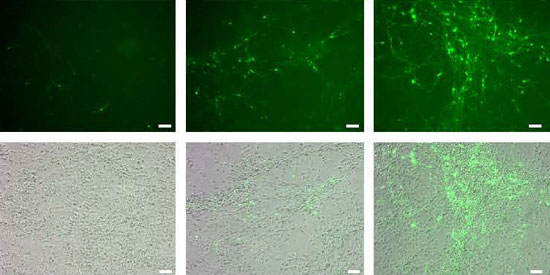 |
Stem cells turn into nerve cells: during a period of three weeks, more and more stem cells transform into immature nerve cells (green). (© Biomaterials)
|
The medical use of stem cells has made great progress in recent years, but important questions remain unanswered: Where do stem cells have to be implanted to maximise their chance of survival? What conditions are required for them to develop into nerve cells and optimally promote the regeneration process? Until now, scientists were only able to assess the survival and development of such stem cells in tissue sections under the microscope, not in living animals. Thus, it was only possible to obtain snapshots of the months-long process by which a stem cell develops into a nerve cell.
|
With the new method it is now possible to observe the entire process in the living brain. The scientists hope to obtain new knowledge that will lead, for instance, to the development of better treatments for stroke. The Research Group led by Mathias Hoehn at the Max Planck Institute for Metabolic Research modified stem cells in such a way that they become detectable for imaging modalities only in specific phases of their development. To this end, they inserted genes for three optical imaging reporters into the genome of the stem cells: the protein ferritin, which serves as a contrast agent in magnetic resonance imaging (MRI); the enzyme luciferase, which stimulates stem cells in the brain to emit light; and the fluorescent protein GFP. Luciferase produces light of such intensity in the living brain that it can be recorded with sensitive cameras even through thick tissue layers. GFP, by contrast, renders the cells visible for subsequent examination under the microscope.
|
Various gene regulators ensure that the genes are activated during different phases. Thus, the stem cells reveal their developmental state into mature neurons by emitting light only when the preselected gene is activated. Using a highly sensitive camera, the researchers are thereby able to follow the development of stem cells into mature nerve cells. At the same time, they can identify the cells with the help of the self-produced contrast agent ferritin in magnetic resonance imaging scans and, for example, observe their migration to the site of a stroke.
|
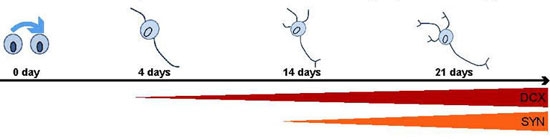 |
Gene regulators indicate the developmental state of cells: the DCX promotor is already getting active in immature cells; SYN only in mature neurons which form snyapses with other cells. (© Biomaterials)
|
Using this method, the scientists discovered that stem cells develop into immature nerve cells just four days after being implanted into a mouse brain. “Over a period of four weeks, more and more stem cells develop into such nerve cells. After three months, mature nerve cells have formed in the brain,” says Annette Tennstaedt from the Max Planck Institute in Cologne. The cells are already electrically active and therefore functional, as their cooperation partners, a team of researchers headed by Peter Kloppenburg at Cologne University have shown.
|
The method developed by the Cologne-based researchers will enable scientists to study the fate of transplanted stem cells more closely. For example, it will be possible to investigate in the mouse brain how stem cells migrate from their site of origin to damaged tissue, where they then replace dead cells after a stroke. The new method can also be applied to nerve cells with very specific functions, for example the replacement of dopaminergic nerve cells in Parkinson’s disease. It will also be possible to reduce the number of experimental animals significantly, as the same animal can be studied again and again at different times.
|