Dec 01, 2017 |
Study sheds light on turbulence in astrophysical plasmas
|
(Nanowerk News) Plasmas, gas-like collections of ions and electrons, make up an estimated 99 percent of the visible matter in the universe, including the sun, the stars, and the gaseous medium that permeates the space in between. Most of these plasmas, including the solar wind that constantly flows out from the sun and sweeps through the solar system, exist in a turbulent state. How this turbulence works remains a mystery; it’s one of the most dynamic research areas in plasma physics.
|
Now, two researchers have proposed a new model to explain these dynamic turbulent processes.
|
The findings, by Nuno Loureiro, an associate professor of nuclear science and engineering and of physics at MIT, and Stanislav Boldyrev, a professor of physics at the University of Wisconsin at Madison, are reported in the Astrophysical Journal ("Collisionless Reconnection in Magnetohydrodynamic and Kinetic Turbulence"). The paper is the third in a series this year explaining key aspects of how these turbulent collections of charged particles behave.
|
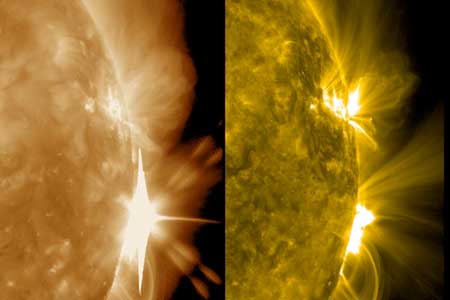 |
Magnetic reconnection is a complicated phenomenon that Nuno Loureiro, an associate professor of nuclear science and engineering and of physics at MIT, has been studying in detail for more than a decade. To explain the process, he gives a well-studied example: “If you watch a video of a solar flare” as it arches outward and then collapses back onto the sun’s surface, “that’s magnetic reconnection in action. It’s something that happens on the surface of the sun that leads to explosive releases of energy.” Loureiro’s understanding of this process of magnetic reconnection has provided the basis for the new analysis that can now explain some aspects of turbulence in plasmas. (Image: NASA)
|
“Naturally occurring plasmas in space and astrophysical environments are threaded by magnetic fields and exist in a turbulent state,” Loureiro says. “That is, their structure is highly disordered at all scales: If you zoom in to look more and more closely at the wisps and eddies that make up these materials, you’ll see similar signs of disordered structure at every size level.” And while turbulence is a common and widely studied phenomenon that occurs in all kinds of fluids, the turbulence that happens in plasmas is more difficult to predict because of the added factors of electrical currents and magnetic fields.
|
“Magnetized plasma turbulence is fascinatingly complex and remarkably challenging,” he says.
|
Magnetic reconnection is a complicated phenomenon that Loureiro has been studying in detail for more than a decade. To explain the process, he gives a well-studied example: “If you watch a video of a solar flare” as it arches outward and then collapses back onto the sun’s surface, “that’s magnetic reconnection in action. It’s something that happens on the surface of the sun that leads to explosive releases of energy.” Loureiro’s understanding of this process of magnetic reconnection has provided the basis for the new analysis that can now explain some aspects of turbulence in plasmas.
|
Loureiro and Boldyrev found that magnetic reconnection must play a crucial role in the dynamics of plasma turbulence, an insight that they say fundamentally changes the understanding of the dynamics and properties of space and astrophysical plasmas and “is indeed a conceptual shift in how one thinks about turbulence,” Loureiro says.
|
Existing hypotheses about the dynamics of plasma turbulence “can correctly predict some aspects of what is observed,” he says, but they “lead to inconsistencies.”
|
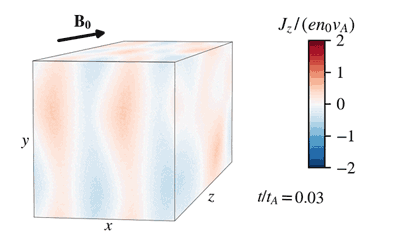 |
Simulation conducted by MIT student Daniel Groselj.
|
Loureiro worked with Boldyrev, a leading theorist on plasma turbulence, and the two realized “we can fix this by essentially merging the existing theoretical descriptions of turbulence and magnetic reconnection,” Loureiro explains. As a result, “the picture of turbulence gets conceptually modified and leads to results that more closely match what has been observed by satellites that monitor the solar wind, and many numerical simulations.”
|
Loureiro hastens to add that these results do not prove that the model is correct, but show that it is consistent with existing data. “Further research is definitely needed,” Loureiro says. “The theory makes specific, testable predictions, but these are difficult to check with current simulations and observations.”
|
He adds, “The theory is quite universal, which increases the possibilities for direct tests.” For example, there is some hope that a new NASA mission, the Parker Solar Probe, which is planned for launch next year and will be observing the sun’s corona (the hot ring of plasma around the sun that is only visible from Earth during a total eclipse), could provide the needed evidence. That probe, Loureiro says, will be going closer to the sun than any previous spacecraft, and it should provide the most accurate data on turbulence in the corona so far.
|
Collecting this information is well worth the effort, Loureiro says: “Turbulence plays a critical role in a variety of astrophysical phenomena,” including the flows of matter in the core of planets and stars that generate magnetic fields via a dynamo effect, the transport of material in accretion disks around massive central objects such as black holes, the heating of stellar coronae and winds (the gases constantly blown away from the surfaces of stars), and the generation of structures in the interstellar medium that fills the vast spaces between the stars. “A solid understanding of how turbulence works in a plasma is key to solving these longstanding problems,” he says.
|
“This important study represents a significant step forward toward a deeper physical understanding of magnetized plasma turbulence,” says Dmitri Uzdensky, an associate professor of physics at the University of Colorado, who was not involved in this work. “By elucidating deep connections and interactions between two ubiquitous and fundamental plasma processes — magnetohydrodynamic turbulence and magnetic reconnection — this analysis changes our theoretical picture of how the energy of turbulent plasma motions cascades from large down to small scales.”
|
He adds, “This work builds on a previous pioneering study published by these authors earlier this year and extends it into a broader realm of collisionless plasmas. This makes the resulting theory directly applicable to more realistic plasma environments found in nature. At the same time, this paper leads to new tantalizing questions about plasma turbulence and reconnection and thus opens new directions of research, hence stimulating future research efforts in space physics and plasma astrophysics.”
|