Posted: June 16, 2009 |
Nonstick gold surfaces and laser-safe gold nanoposts aids trapping of biomolecules |
(Nanowerk News) Biophysicists long for an ideal material—something more structured and less sticky than a standard glass surface—to anchor and position individual biomolecules. Gold is an alluring possibility, with its simple chemistry and the ease with which it can be patterned. Unfortunately, gold also tends to be sticky and can be melted by lasers. Now, biophysicists at JILA have made gold more precious than ever—at least as a research tool—by creating nonstick gold surfaces and laser-safe gold nanoposts, a potential boon to laser trapping of biomolecules.
|
JILA is a joint institute of the National Institute of Standards and Technology (NIST) and the University of Colorado at Boulder.
|
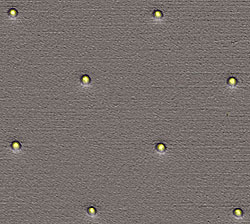 |
The gold posts in this colorized micrograph, averaging 450 nanometers in diameter, are used to anchor individual biomolecules such as DNA for studies of their mechanical properties. The background surface is glass coated with a protein to prevent unwanted sticking. (Image: D.H. Paik/JILA)
|
JILA’s successful use of gold in optical-trapping experiments, reported in Nano Letters,* could lead to a 10-fold increase in numbers of single molecules studied in certain assays, from roughly five to 50 per day, according to group leader Tom Perkins of NIST. The ability to carry out more experiments with greater precision will lead to new insights, such as uncovering diversity in seemingly identical molecules, and enhance NIST’s ability to carry out mission work, such as reproducing and verifying piconewton-scale force measurements using DNA, Perkins says. (A one-kilogram mass on the Earth’s surface exerts a force of roughly 10 newtons. A piconewton is 0.000 000 000 001 newtons. See “JILA Finds Flaw in Model Describing DNA Elasticity”)
|
Perkins and other biophysicists use laser beams to precisely manipulate, track and measure molecules like DNA, which typically have one end bonded to a surface and the other end attached to a micron-sized bead that acts as a “handle” for the laser. Until now, creating the platform for such experiments has generally involved nonspecifically absorbing fragile molecules onto a sticky glass surface, producing random spacing and sometimes destroying biological activity. “It’s like dropping a car onto a road from 100 feet up and hoping it will land tires down. If the molecule lands in the wrong orientation, it won’t be active or, worse, it will only partially work,” Perkins says.
|
|
Ideally, scientists want to attach biomolecules in an optimal pattern on an otherwise nonstick surface. Gold posts are easy to lay down in desired patterns at the nanometer scale. Perkins’ group attached the DNA to the gold with sulfur-based chemical units called thiols (widely used in nanotechnology), an approach that is mechanically stronger than the protein-based bonding techniques typically used in biology ("Integrating a High-Force Optical Trap with Gold Nanoposts and a Robust Gold-DNA Bond"). The JILA scientists used six thiol bonds instead of just one between the DNA and the gold posts. These bonds were mechanically strong enough to withstand high-force laser trapping and chemically robust enough to allow the JILA team to coat the unreacted gold on each nanopost with a polymer cushion, which eliminated undesired sticking. “Now you can anchor DNA to gold and keep the rest of the gold very nonstick,” Perkins says.
|
Moreover, the gold nanoposts were small enough—with diameters of 100 to 500 nanometers and a height of 20 nanometers—that the scientists could avoid hitting the posts directly with lasers. “Like oil and water, traditionally laser tweezers and gold don’t mix. By making very small islands of gold, we positioned individual molecules where we wanted them, and with a mechanical strength that enables more precise and additional types of studies,” Perkins says.
|