Nanotechnology and the concept of friction
(Nanowerk Primer) Friction is the name given to the force that resists the initiation of sliding motion between two surfaces; it is all around us and it occurs at all length scales. In simple terms: friction is a force that slows things down. This is one reason why you can't have a perpetuum mobile, i.e. a motion that continues indefinitely without any external source of energy, as long as there is any friction.
Contents: Nanotechnology and the concept of friction
1 Why is friction such an issue?
Science notes: Friction basics (key takeaways and vocabulary)
2.4 Superlubricity
Science notes: Friction at the nanoscale (key takeaways and vocabulary)
1.1 What is friction?
Take this everyday example: when a coffee mug rests on a flat table, the kinetic frictional force is zero. There is no force trying to move the mug across the table, so there is no need for a frictional force because there is nothing for the frictional force to oppose. However, if you try to slide the mug across the table then a friction force will come into play and initially resist your push until your pushing force exceeds the frictional force.
In other words, in order to set a stationary object in motion, its static friction must be overcome first.
If your coffee mug rests on a slanted surface, like an inclined ramp, then a frictional force opposing gravity will initially prevent the mug from sliding down. If the ramp is inclined far enough, the frictional force will grow until the point that it is unable to prevent the mug from sliding.
To put it this way: once the static friction has been overcome, the sliding friction sets in and consumes additional energy.
To generate motion in the first place, it is not sliding friction but static friction that must be overcome. Static friction is typically larger than sliding friction and a result of the atomic structure of the contact surfaces locking into place. The surfaces can only free themselves and move against each other once the applied force has reached adequate levels.
In scientific terms: friction between two surfaces is caused by energy loss as atoms from the opposing surfaces smash against each other. In the presence of an attractive or adhesive force the atoms are in intimate contact and much energy is lost in forcing the atoms to slide past each other.
There are four types of friction: static, sliding, rolling, and fluid friction. The last three are also grouped under the term kinetic friction. The first three occur between solid surfaces; fluid friction occurs in liquids and gases.
Static friction acts on objects when they are resting on a surface, for instance when you are standing on a floor.
Sliding friction, which is weaker than static friction, acts on objects when they are sliding over a surface, for instance when a musician's bow is drawn across a violin's strings.
Rolling friction, which is much weaker than sliding friction or static friction, acts on objects when they are rolling over a surface (for instance tires or ball bearings).
Fluid friction acts on objects that are moving through a fluid, like ships in water.
Friction itself isn't good or bad, but it could be too much or too little; it depends on the circumstance in which it occurs. Static friction – in contrast to sliding friction – is often a desired phenomenon.
You definitely want plenty of static friction from your automobile's brakes or clutch. In many other cases, it is essential – if there was no friction at all you couldn't accelerate your car or bicycle since the wheels would simply spin without getting a grip on the road. Static friction also enables us to safely grasp objects. You couldn't write, as the pen would slip off the paper without any resistance. You couldn't even hold the pen, as it would slip out of your hand when you try to grab it.
Rather than only trying to reduce friction, we also use friction to our advantage: on icy roads, sand increases friction and enables us to walk more safely. In the automobile industry, brake pads are engineered to provide a high degree of friction.
1.2 Friction in Nature
In Nature, there are no completely frictionless environments: even in deep space, tiny particles may interact and cause friction. Friction processes can be observed at all scales and dimensions.
Scientists and engineers often are inspired by the efficiency and durability of natural systems and are trying to duplicate them for man-made systems (a field called biomimetics).
Millions of years of evolution have allowed Nature to come up with some pretty impressive strategies for minimizing or maximizing friction depending on the required function. Numerous organisms have developed solutions to provide ultra low friction in the case of lubricated systems; ultra high friction in the case of adhesives; or in some cases even controlled adaptable friction performance (see: International Journal of Design Engineering, "Friction in nature").
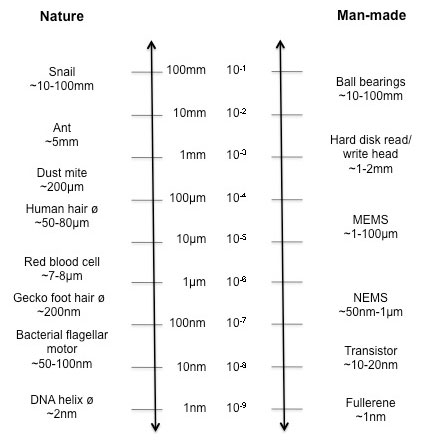
We talk about adhesion when the friction between two surfaces is so strong that it provides a bonding force. Adhesion is a general term for several types of relatively short-range, attractive forces that act between solid surfaces, including the van der Waals force, electrostatic force, chemical bonding, and the capillary force due to the condensation of water at the surface.
Adhesion in natural systems is achieved in different ways, depending mainly on the evolutionary processes that created them.
One famous example is the gecko adhesive effect. Even without secretion or glue, Nature's dry adhesive systems are very reliable as the contact is split into numerous very tiny fibrillar features, creating a nanopatterned surface. Geckos have foot pads that are composed of millions of tiny branching hairs at their end, called setae (3-130 micrometers in length), splitting into even smaller spatulae (about 200 nanometers in both width and length). This structural design produces the high adhesion and friction forces between gecko and a surface via molecular interaction forces. That's how geckos manage to climb vertical walls or walk upside-down on ceilings.
The sandfish of the Sahara (Scincus scincus) provides a great example for the opposite effect: the nano-ridges together with the nano-spikes on this reptile's scales have been evolved to reduce skin friction (see: "Sandfish inspires engineering"). This allows it to 'swim' effortless under the loose sand of a dune.
Other examples that illustrate Nature's friction designs:
• The Notonecta glauca bug has a surface covered by high-density hairs, which allows it to keep an air layer under water. This enables this insect to move nimbly and swiftly through water by reducing the drag on its surface.
• After a rain, the cupped leaf of a pitcher plant becomes a almost frictionless surface. Sweet smelling and elegant, the carnivore attracts ants, spiders, and even little frogs. One by one, they slide to their doom.
• All fish species are covered with mucus to reduce drag. Researchers have found that slime secretion can reduce drag by 50% to 60%.
• Snails have evolved an ingenious way to use mucus: as an adhesive (to stick to walls) as well as a lubricant (to move).
• Sharkskin is covered in small scales (0.2-0.5 mm) with very fine longitudinal ridges that also reduce hydrodynamic drag (drag is a specific case of friction at the liquid interface).
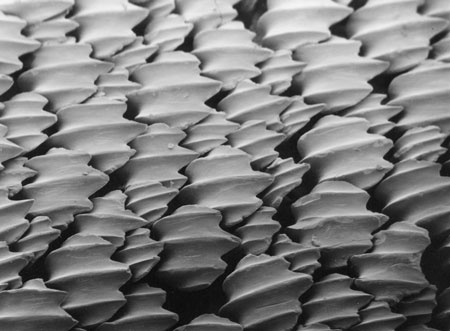
Interesting frictional characteristics have also formed in the non-biological world. Here, control of friction is not part of evolution but these systems still have potentially interesting properties from a technical point of view.
One prominent example is ice friction, which is notoriously low, as you well know if you ever slipped and fell on an icy surface. Fundamental research on ice friction has practical applications for materials being in contact with ice, like skies, skates or tires.
The question why ice is slippery has engaged scientists for over a century until researchers have shown that the slipperiness of ice is a consequence of the ease with which the topmost water molecules can roll over the ice surface (see: The Journal of Physical Chemistry Letters, "Molecular Insight into the Slipperiness of Ice").
Scientists believe that although liquid water at the ice surface does reduce sliding friction on ice, this liquid water is not melted by pressure but by frictional heat produced during sliding. Interestingly, with sinking temperatures the ice surface transforms from an extremely slippery surface at typical winter sports temperatures (it is most slippery at minus 7°C), to a surface with high friction at minus 100°C.