Posted: Jun 06, 2016 |
A new low-defect method to nitrogen dope graphene resulting in tunable bandstructure
(Nanowerk News) An interdisciplinary team of scientists at the U.S. Naval Research Laboratory (NRL), Electronics Science and Technology and Materials Science and Technology Divisions, has demonstrated hyperthermal ion implantation (HyTII) as an effective means of substitutionally doping graphene -- a hexagonally-arranged single-atomic thickness carbon sheet -- with nitrogen atoms. The result is a low-defect film with a tunable bandstructure amenable to a variety of device platforms and applications.
|
The results of this research are reported in ACS Nano ("Nitrogen-Doped Graphene and Twisted Bilayer Graphene via Hyperthermal Ion Implantation with Depth Control"), which details the fabrication method and efficient doping energy range, and Physical Review B: Rapid Communications ("Electronic transport and localization in nitrogen-doped graphene devices using hyperthermal ion implantation"), which details the electronic transport properties of devices made from the films.
|
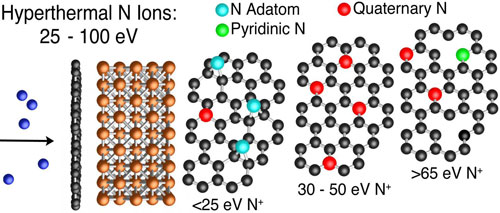 |
Schematic displaying the HyTII process depicting nitrogen (N+) incident with a graphene-on-copper (Cu) sample. The three-graphene flake schematics depict the type of modification resulting from N-HyTII processing over the labeled energy range. (Image: US Naval Research Laboratory)
|
The research shows that the HyTII method delivers a high degree of control including doping concentration and, for the first time, demonstrates depth control of implantation by doping a single monolayer of graphene in a bilayer graphene stack. This further demonstrates that the resulting films have high-quality electronic transport properties that can be described solely by changes in bandstructure rather than the defect-dominated behavior of graphene films doped or functionalized using other methods.
|
"Since the discovery that a single atomic layer of sp2 bonded carbon atoms, termed graphene, could be isolated from bulk graphite, a plethora of remarkable electronic and spintronic properties have emerged," said Dr. Cory Cress, materials research engineer, NRL. "However, few applications are forthcoming because graphene lacks a bandgap and its doping is difficult to control, rendering graphene devices competitive only for highly-specialized device technologies."
|
Doping or chemical functionalization may add a usable transport gap. However, these methods tend to produce films that are plagued by unintentional defects, have low stability, or non-uniform coverage of dopants and functional groups, which all greatly limit their usefulness and degrade the intrinsic desirable properties of the graphene film.
|
As an alternative, NRL scientists leveraged their radiation-effects background to develop a hyperthermal ion implantation system with the necessary precision and control to implant nitrogen (N+) into graphene achieving doping via direct substitution.
|
"After many months of developing the system, the feasibility of the technique really depended on the first experiment," Cress said. "In our study, we determined the range of hyperthermal ion energies that yielded the highest fraction of nitrogen doping, while minimizing defects, and we were successful in confirming the inherent depth control of the HyTII process."
|
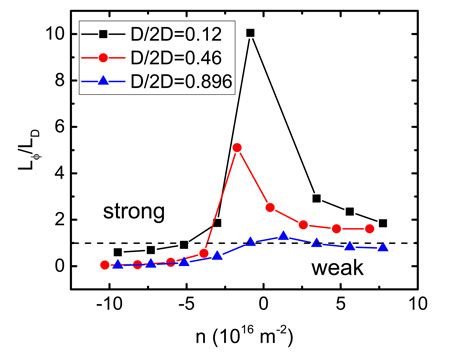 |
Graphical Plot: This plot displays the characteristic lengths of the system as a function of carrier density for three different implantation doses. The dotted line separates the weak localization regime (semiconductor) from the strong localization regime (insulator). As dose (measured by the Raman D/2D ratio), therefore nitrogen content, increases, the transition from weak to strong localization is suppressed near the charge neutrality point. These analyses, along with other measurements, indicate that the nitrogen-doped films are low-defect and that the bandstructure is tunable. (Image: US Naval Research Laboratory)
|
To achieve the latter, the scientists implemented a bilayer graphene material system comprising a layer of natural graphene, with mostly carbon-12 (12C) atoms, layered on graphene synthesized with greater than 99 percent carbon-13 (13C). This bilayer material provided a means to identify which layer they were modifying when analyzed with Raman spectroscopy.
|
Devices made from films processed with N+ in the range of optimal doping show a transition from strong to weak localization that depends on implantation dose, indicating the implanted nitrogen's ability to alter the intrinsic properties of the film. As further evidenced by the high electronic quality of the implanted devices over similar adatom-doped devices, the scientists found that the temperature dependence can be fit by a model that takes into account both band effects due to the substitutional doping and insulator-like effects due to defect formation, with the band effects observed to be the dominant component.
|
Surprisingly, the researchers found that a higher amount of nitrogen doping prevents the crossover to insulating behavior near the charge neutrality point. Defects appear to dominate the behavior only at large implantation energies, where defects are more probable, further demonstrating the differences between true-doped films and previous defective/doped films.
|
"Our measurements of these devices strongly indicate that we have finally fabricated a graphene film with a tunable bandgap, low defect density, and high stability," explains Dr. Adam L. Friedman, research physicist, NRL. "We therefore hypothesize that HyTII graphene films have great potential for electronic or spintronic applications for high-quality graphene where a transport or bandgap and high carrier concentration are desired."
|