Nov 27, 2023 |
CRISPR enhanced nanotweezers for ultra-precise DNA manipulation and detection
(Nanowerk News) Since the concept of optical trapping was first proposed in the 1960s and 1970s by Arthur Ashkin, researchers have pursued innovative techniques to precisely manipulate and analyze individual biomolecules like DNA. Such capabilities would revolutionize genetics, molecular biology, and medicine by enhancing our understanding of biological systems and enabling ultra-sensitive biosensing. However, progress has been hindered by the difficulty of selectively capturing nanoscale particles in liquid and identifying them.
|
Now, scientists from Shenzhen University have developed “CRISPR-powered optothermal nanotweezers” (CRONT) that can trap specific DNA strands within a solution and detect single-base differences. This breakthrough combines the specificity of CRISPR-based DNA targeting with the precision manipulation of optothermal traps.
|
Their findings have been published in Light: Science & Applications ("CRISPR-powered optothermal nanotweezers: Diverse bio-nanoparticle manipulation and single nucleotide identification").
|
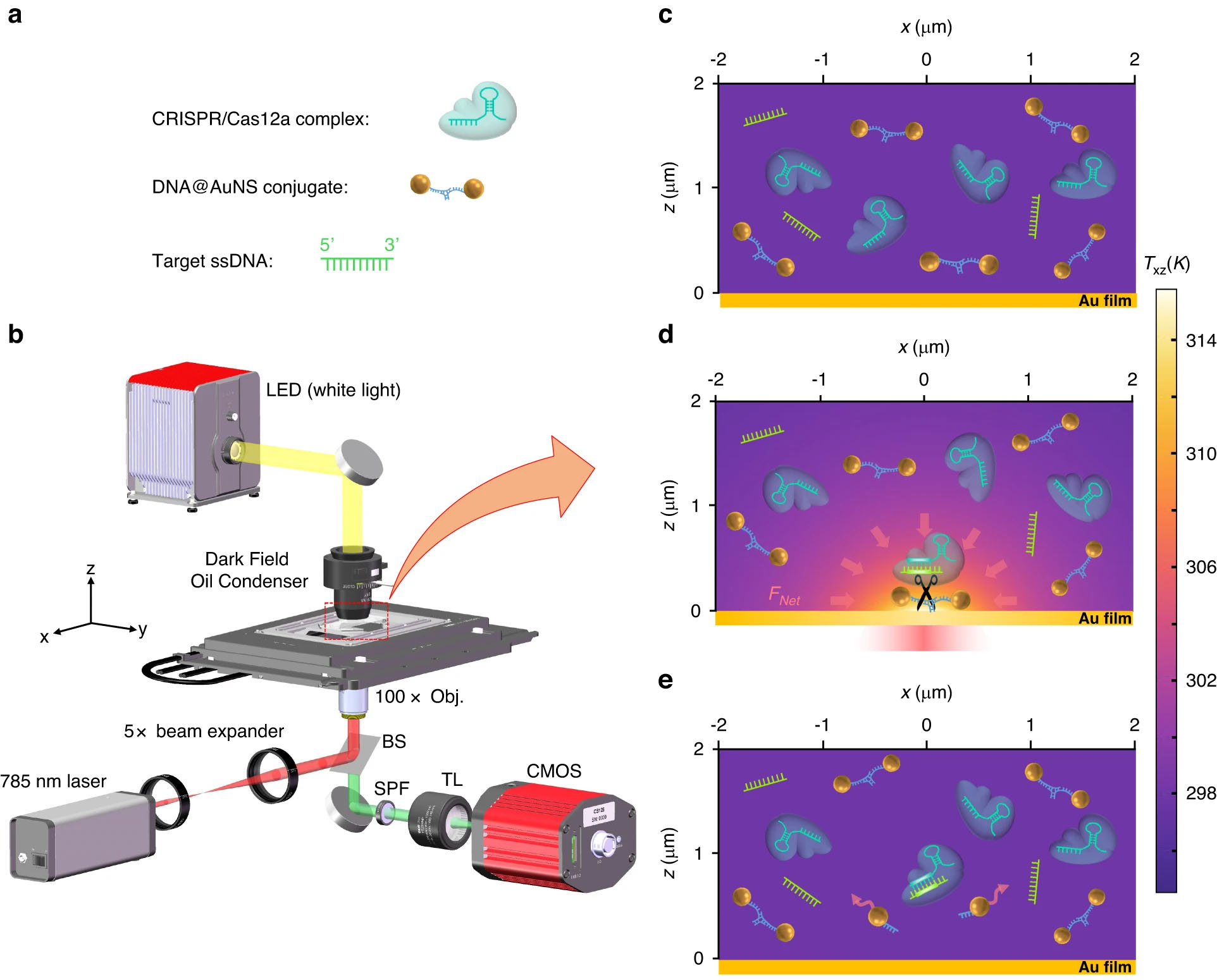 |
Working principle of CRONT. a The diagrammatic sketch of the three components in the solution: DNA@AuNS conjugate, CRISPR/Cas12a complex, and target ssDNA. b Optical setup, the BS, SPF, and TL are beam splitter, short pass filter, and tube lens (f =200 mm), respectively. Additional details of the setup are provided in the Materials and Methods section. c Dispersion of the three components in the solution without optical heating. d Optothermal net force (FNet) induced migration and DNA@AuNS conjugate cleavage upon optical heating, the heating laser power is 0.5 mW. e Observation of the cleavage after the optical heating is switched off (© Light: Science & Applications)
|
Optical trapping tools like optical and optothermal tweezers have long attracted interest across science and engineering fields for their versatility in manipulating microscopic objects without contact. They use a highly focused laser beam to generate forces that can trap small particles.
|
But conventional optical tweezers provide insufficient force and selectivity to analyze single biomolecules in liquid, especially DNA, restricting their use. This has motivated alternative schemes leveraging nanoplasmonics, electrical fields, and temperature gradients. The latter approach, termed optothermal tweezers, induces strong thermophoresis and fluid motion that gathers molecules. However, until now these techniques lacked the means to definitively identify the trapped substances.
|
In parallel, CRISPR gene editing has emerged as an extremely precise method for targeting DNA sequences by exploiting sequence-specific guide RNAs. Although powerful for biosensing, CRISPR techniques have traditionally depended upon slow, random diffusion to bring sparse target DNA strands in contact with CRISPR enzyme complexes in solution. This bottleneck has limited detection sensitivity and hindered CRISPR's use for rapidly screening low abundance mutants.
|
The new work unites these domains by integrating CRISPR probing into optothermal tweezers for the first time. The researchers created CRONT chips with thin gold heating films to generate optothermal manipulation forces within a microscopic analysis chamber. This lets them actively gather and trap targeted DNA-linked gold nanoparticles using CRISPR sequences. Monitoring nanoparticle integrity then indicates DNA cleavage via CRISPR, confirming the presence and exact sequence of complementary target DNA strands down to femtomolar concentrations. Using single DNA-gold probes, CRONT can even pinpoint single nucleotide variations.
|
Such capabilities would be transformative for genetics, synthetic biology, and precision diagnostics. As the authors highlight, CRONT could serve as a versatile biosensing platform, support elucidating complex biological pathways by tracking the interactions and reactions of individual molecules in real-time, as well as enable DNA-targeted manipulation for advanced optothermal sorting systems. Early disease screens, rapid identification of emerging viral mutations, and on-site environmental DNA testing are just some valuable applications.
|
Powering these functions is an ingenious yet elegant system design. An infrared laser focused on the gold film creates a hotspot that heats the surrounding liquid to an optimal 37 °C for CRISPR enzymes. This also generates fluid flows and thermophoretic forces that actively accumulate DNA-gold nanoparticles within the irradiated region. Hybridizing particles with a capture sequence binds them to a CRISPR protein-RNA complex programmed to cut only sequence-matched DNA. Infused target DNA then drives sequence-specific cleavage and release of the DNA linkers. By briefly switching off the laser, the separated gold particles freely diffuse away, signaling successful target detection. Sensitive dark-field microscopy detects single cleavage events.
|
Through experimental analysis and simulations, the group demonstrated that CRONT’s combined optothermal manipulation and localized heating enhances CRISPR reactions even in tiny 10 microliter volumes. This enables robust detection despite limited DNA while reducing reagent needs and assay time. Using this system with Cas12a enzymes, the team could identify single-stranded DNA from monkeypox and SARS-CoV-2 down to 25 attomoles within 2 minutes - orders of magnitude faster than conventional methods. They also confirmed CRONT can distinguish single nucleotide mutations between SARS-CoV-2 variants, with excellent specificity when screening multiple sequences.
|
While still an initial proof-of-concept, researchers will likely optimize CRONT’s capabilities further in the coming years. Possible upgrades include parallel sensing with multi-beam heating to increase throughput, plus software automation for ease-of-use. The authors also suggest that as well as studying biological behaviors and recognizing pathogens or mutations, CRONT might directly guide CRISPR editing, gene regulation, and nucleic acid assembly by dynamically manipulating target DNA with high spatiotemporal control. Paired with appropriate surface chemistry, more generalized molecular trapping architectures leveraging thermophoresis could also be possible.
|
Through decades of interdisciplinary advances, the technologies needed to probe biology on the nanoscale with exquisite accuracy have gradually come within reach. As tools like these new programmable optothermal tweezers mature to match our ambitions, a new era of single molecule bioengineering seems imminent.
|