Mar 28, 2024 |
Room-temperature 2D magnet: Electronic-structure insights
(Nanowerk News) With the help of data from the Advanced Light Source (ALS), researchers found that small changes in how electron spins interact with each other can make a big difference in the magnetic transition temperatures of 2D magnets.
|
The findings are published in Nano Letters ("Electronic structure of above-room-temperature van der Waals ferromagnet Fe3GaTe2").
|
Understanding the factors affecting magnetic transition temperatures can help create better magnetic materials for information storage, sensors, medical imaging, and energy-efficient computing.
Artwork depicting the use of angle-resolved photoemission to reveal the electronic structure (upper right inset) of iron gallium telluride (Fe3GaTe2), a 2D material that exhibits ferromagnetism at room temperature. Blue arrows represent magnetic moments (spins) aligning perpendicular to the plane of the material.
|
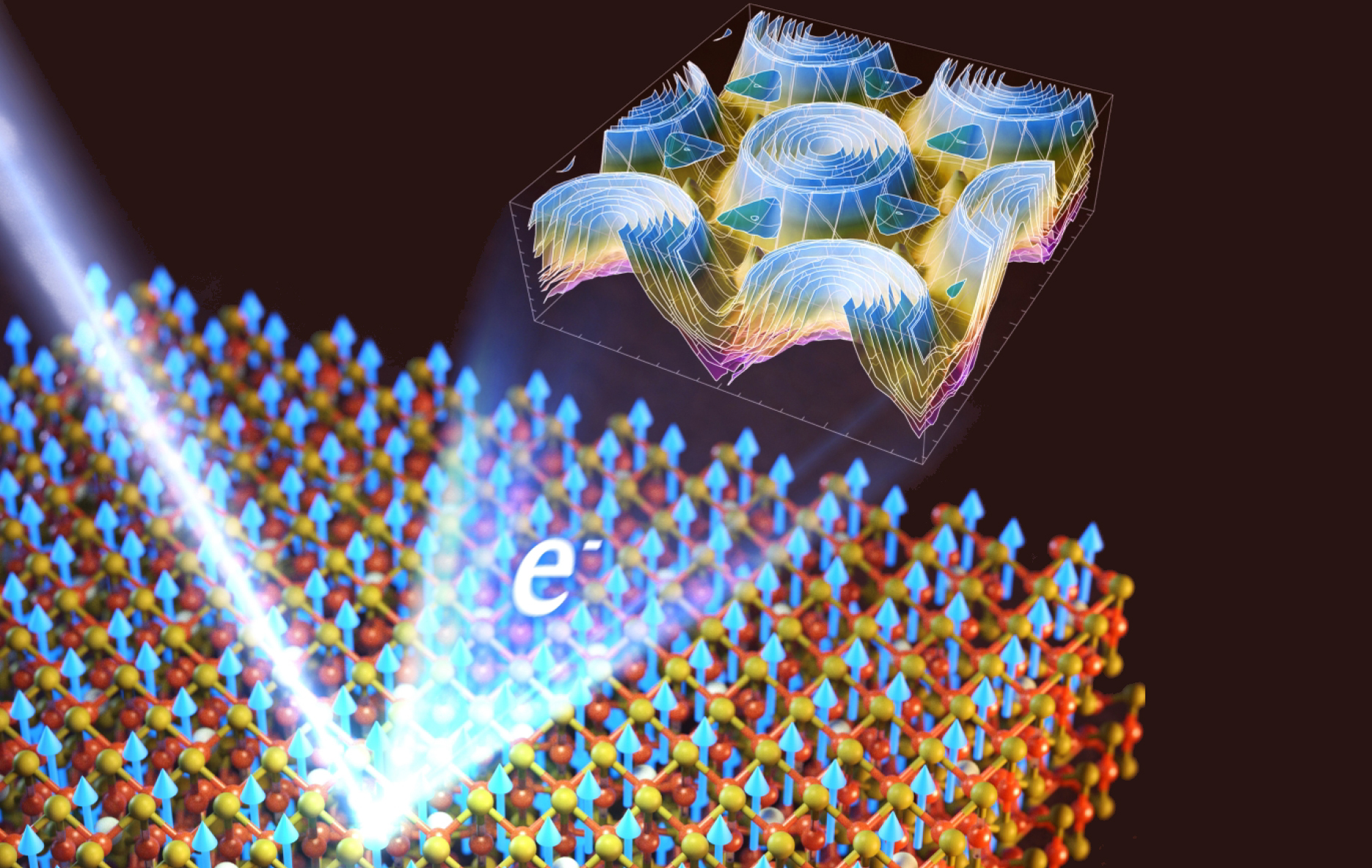 |
Artwork depicting the use of angle-resolved photoemission to reveal the electronic structure (upper right inset) of iron gallium telluride (Fe3GaTe2), a 2D material that exhibits ferromagnetism at room temperature. Blue arrows represent magnetic moments (spins) aligning perpendicular to the plane of the material. (Image: Advanced Light Source)
|
Toward atomically thin magnets
|
“Two-dimensional” (2D) magnetic materials – just a few atomic layers thick – have attracted a lot of interest recently for several reasons: they are highly tunable, they can be stacked into heterostructures for more functionality, they can be used for energy-efficient computing and data-storage applications, and they can deepen our fundamental understanding of magnetism itself.
|
Unfortunately, many of these materials are not very stable in air and their magnetic transition temperatures are much lower than room temperature. As a first step toward designing materials with more desirable characteristics, scientists need to understand how the materials become magnetic and what factors can improve their properties. Here, researchers used angle-resolved photoemission spectroscopy (ARPES) and computational tools to understand the inner workings of 2D magnetic materials.
|
Comparing “sister” materials
|
Iron gallium telluride (Fe3GaTe2) is a 2D ferromagnet with a record-breaking magnetic transition temperature (~370 K) and a tendency for its magnetic alignment to be perpendicular to the surface—both useful features for spintronics applications. Its closely related “sister” material, iron germanium telluride (Fe3GeTe2), is a well-studied 2D magnetic material with nearly the same crystal structure but a much lower transition temperature (~220 K).
|
To understand how a simple change from germanium to gallium (next to each other in the periodic table) can improve the magnetic transition temperature by such a large margin, the researchers approached the question from an electronic-structure point of view, with ARPES and density functional theory (DFT) calculations.
|
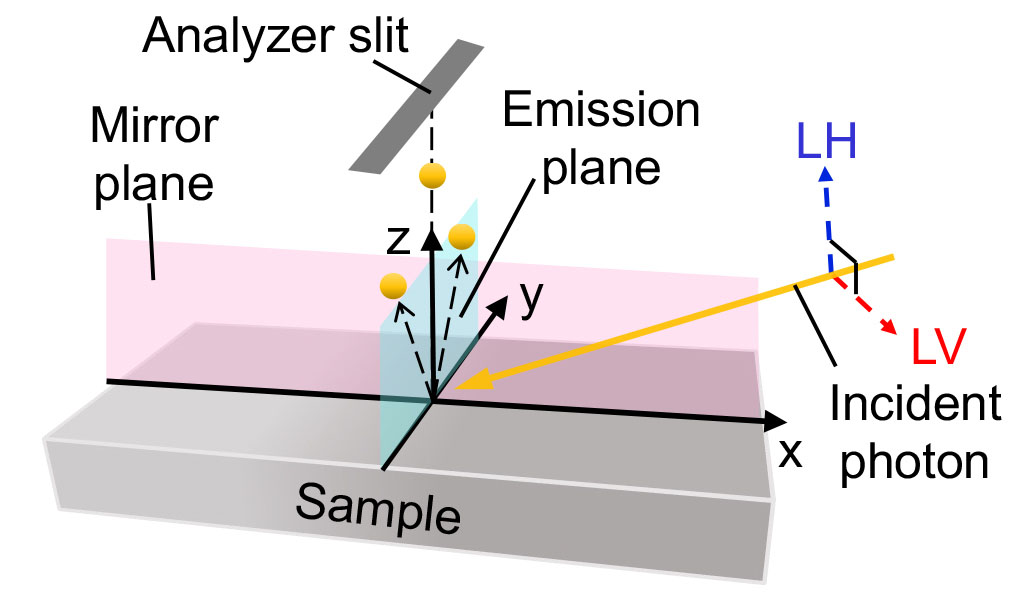 |
Schematic of the polarization geometry of the ARPES measurement. Linear horizontal (LH) and linear vertical (LV) polarizations are defined with respect to the synchrotron light source. (Image: Advanced Light Source)
|
High-resolution ARPES at the ALS
|
The researchers had previously investigated the electronic structures of Fe3GeTe2 using ARPES at ALS Beamline 10.0.1 and DFT calculations. That study revealed that the magnetic anisotropy energy (MAE)—a parameter that quantifies how hard it is to change spin direction—is the key element affecting the magnetic transition temperature of Fe3GeTe2. In this study, the team similarly used ARPES and DFT at ALS Beamlines 4.0.3 and 10.0.1 to study Fe3GaTe2.
|
The success of the work relied on the high-resolution ARPES measurements that both beamlines provide, enabling the discrimination of multiple electronic bands with close energy scales. Furthermore, the ability to perform photon energy- and polarization-dependent experiments enabled them to unveil intricate orbital characteristics, thus facilitating a precise analysis of the electronic structure.
|
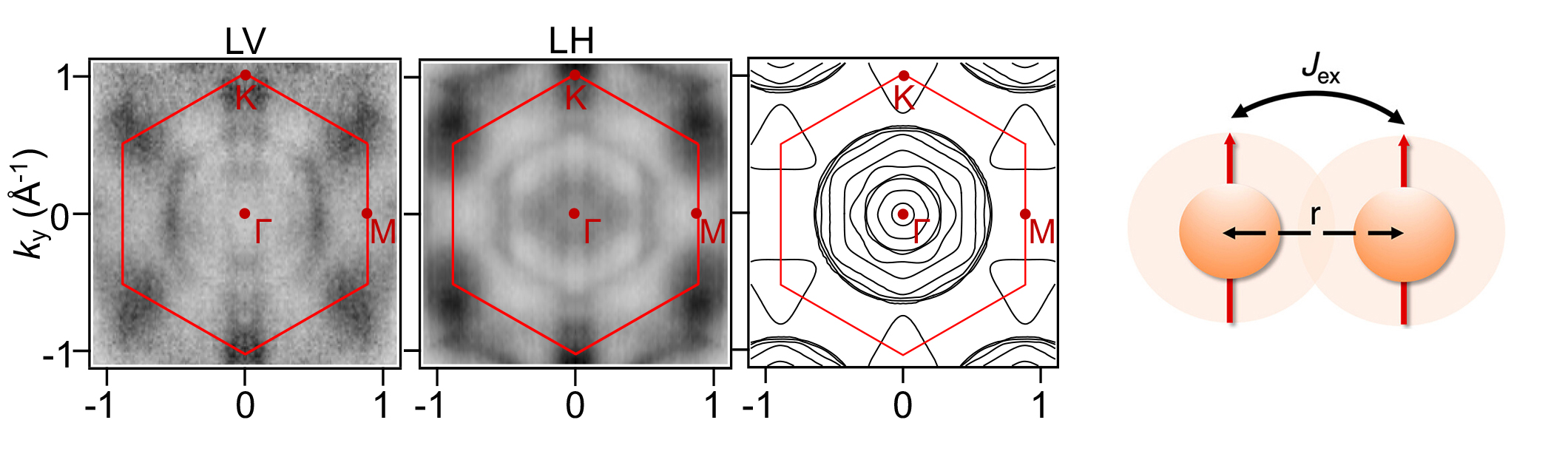 |
ARPES panels on left show the Fermi surface of Fe3GaTe2 at 90 eV, acquired using vertical (LV) and linear horizontal (LH) polarized light. The third panel displays the Fermi surface calculated by DFT. The results establish a consistent correspondence between the measured band structure and theoretical calculations, and enabled careful analysis of the contributions of parameters such as the Heisenberg exchange interaction (Jex) to the development of ferromagnetic ordering in Fe3GaTe2. (Image: Advanced Light Source)
|
The Heisenberg exchange effect
|
Surprisingly, despite the large difference in the transition temperatures of the sister materials, the electronic-structure differences were not very dramatic, indicating that the improved transition temperature of Fe3GaTe2 must have a more subtle origin. Using the ARPES data to set boundary conditions, the researchers calculated values for the MAE as well as the Heisenberg exchange interaction (Jex)—a parameter that quantifies how strongly a pair of spins interact with each other. The results indicated that a small change in Jex due to the slight change in lattice parameters from replacing Ge with Ga can have a significant impact on the magnetic transition temperature.
|
In the future, the researchers plan to explore the doping dependence of the magnetic and transport properties of Fe3GaTe2, as well as the effects of thinning it down to a few atomic layer thicknesses to further improve their understanding of 2D magnetism.
|