Nov 21, 2011 |
Tunnelling electrons under the microscope
|
(Nanowerk News) The team led by ETH Zurich Professor Ursula Keller proved three years ago that so-called tunnelling ionization in helium atoms takes place without any appreciable time delay. They have now shown this is also true for more complex atoms. However, there are several significant effects that need to be taken into account here.
|
Laser pulses can generate powerful electrical fields that detach electrons from an atom. From a quantum mechanical viewpoint, this is equivalent to tunnelling ionisation, i.e. electrons overcome the potential barrier. Using helium atoms, ETH Zurich Professor Ursula Keller and her team showed three years ago that this process, contrary to all assumptions, takes place with almost no time delay. The team, which carries out research in the area known as ultra-fast laser physics, achieved this by using the "atto-clock" which they developed. At that time, its use enabled measurements in the range from one trillionth to one millionth of a millionth of a millionth of a second, i.e. between one femtosecond and one attosecond (1 femtosecond = 10-15 s, 1 attosecond= 10-18 s).
|
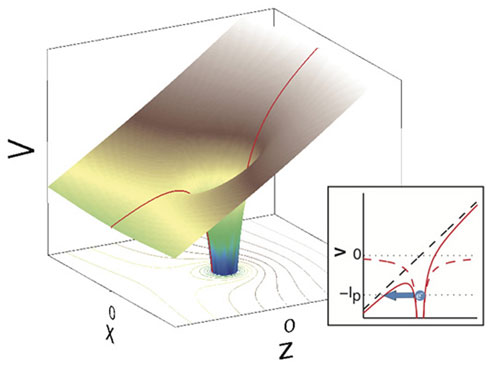 |
Three-dimensional tunnelling by an electron, induced by the electrical field of a laser: the potential of the atom (located at z=x=0) is altered by the laser field running in the z direction. The one-dimensional view in the small image, x=0, shows when the electron can tunnel (blue arrow). (Image: Ursula Keller's research group/ETH Zurich)
|
Discrepancy between theory and measurement
|
The team recently performed new experiments with the more complex argon atom and discovered that the simulation of the expected tunnelling ionisation did not agree with the empirical data. When the researchers tried to explain why the theory and the experiment produced different results, they discovered that the tunnelling effect with the more complex argon atom cannot be considered one-dimensionally, unlike in the case of the helium atom. The researchers needed to include three effects to describe the tunnelling effect correctly: firstly, they had to observe the tunnelling effect in two dimensions; secondly, they needed to take into account the forces acting in the atom; and thirdly, they had to allow for the shift in the energy level when the electrical field was switched on. This allowed the team to successfully characterise the effects known as tunnelling geometry and to show where and when electrons tunnel, and which elements affect this process.
|
The functioning of tunnelling geometry is analogous to topographical maps with the contour lines of mountains. It represents the exact "potential landscape" of the energy barriers. In quantum mechanics, particles can tunnel through these "energy barrier mountains" without a "real" tunnel being formed. It involves a purely quantum mechanical effect that does not exist in our macroscopic world, but which plays a key role in all chemical reactions and in the transport of atomic energy and charge.
|
Two-dimensional treatment is decisive
|
Keller's team used its "atto-clock technique" to measure the electron's two-dimensional momentum from the moment when the electron leaves the "tunnel" through the potential barrier. The measurement confirmed the results of the study published in the scientific journal Science in 2008. Electrons "tunnel" almost instantaneously, even in complex atoms. Keller says, "Our results describe a tunnelling geometry that is easily understandable and whose clear, elegant form continues to fully support the quasi-classical tunnel picture."
|
Their study also allowed the scientists to answer the on-going discussion among experts as to whether the so-called Coulomb forces acting on particles must be taken into account in tunnelling ionisation. It was found that the Coulomb effect on its own is not a sufficient explanation. In fact, the simplified model with only one active electron is no longer satisfied even in a comparatively simple atom such as argon. The many other electrons left behind in the atom must also be included in the treatment, because all the electrons significantly affect the tunnelling geometry: The "multi-electron effect", i.e. the way the tunnel-ionised electrons, the electrons left behind in the atom, and the atomic nucleus act on one another during the tunnelling process must also be taken into account. Claudio Cirelli, one of Keller's postdocs and a co-author of the study published in Nature Physics ("Attoclock reveals natural coordinates of the laser-induced tunnelling current flow in atoms"), says, "Danish colleagues working in theoretical physics developed an equation specifically for this purpose with which these forces can be calculated to an approximation." He says that this theory is based on effects already measured independently in other experiments.
|
However, the "multi-electron effects" inside the argon atoms are so fast that the researchers were unable to resolve their dynamics even with the atto-clock. By using refined and even more complex measurement methods, including on molecules, the team hopes to time-resolve the fast "multi-electron effects" and to be able to study as yet unknown electron correlations.
|