Nov 25, 2013 |
Steering electrons along chemical bonds
|
(Nanowerk News) An ionic crystal is a regular arrangement of positively and negatively charged ions in space. A prototype material is the rock salt crystal sodium chloride (NaCl) with elementary units in form of a cube. This cube contains positively charged Na+ ions in which one electron is lacking, and negatively charged Cl- ions with one extra electron (Fig. 1). Another material with this cubic structure is lithium hydride (LiH), consisting of lithium (Li) and hydrogen (H) atoms. In contrast to the ionic rock salt Na+Cl-, counting the charges in LiH gives Li0.5+H0.5-, striking a happy medium between the ionic case Li+H- and the so-called covalent case Li0+H0- in which electrons are shared between lithium and hydrogen.
|
The peculiar behavior of LiH originates from the strong electric forces between all charged particles in the crystal. Electric interactions lead to a spatial arrangement of electrons in which the total electric energy is minimized. Application of an external electric field to the crystal sets the electrons in motion, again strongly influenced by the spatial correlations among all electrons. Electron correlations have been a subject of many theoretical studies while direct experimental insight is mostly lacking.
|
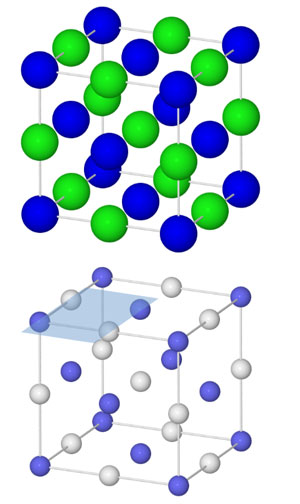 |
Fig. 1: Crystals with rock salt structure. Upper crystal: sodium chloride (NaCl) with blue balls for Na+ ions and green balls for Cl- ions. Lower crystal: lithium hydride (LiH) with small blue balls for Li0.5+ ions and white balls for H0.5- ions. The grey-shaded plane indicates the sectional views shown in Fig.2.
|
A research team at the Max-Born-Institute has now addressed electron correlations by following ultrafast electron motions in space and time, in this way generating ‘maps’ of the electron distribution. In the experiments, electrons are set in motion by a very strong electric field which is provided for the very short time interval of 50 fs (1 fs = 10-15 s) by a strong optical pulse interacting with the LiH material. Then, a 100 fs long x-ray pulse is scattered from the ‘excited’ crystal and images the momentary electron distribution.
|
In the current issue of Physical Review Letters ("Field-Driven Dynamics of Correlated Electrons in LiH and NaBH4 Revealed by Femtosecond X-Ray Diffraction"), Vincent Juvé, Marcel Holtz, Flavio Zamponi, Michael Woerner, Thomas Elsaesser, and Andreas Borgschulte present transient electron distributions, showing an extremely fast shift of electronic charge from the Li0.5+ to the H0.5- ions over a distance of 0.2 nm. This totally unexpected result means that the material becomes more ionic upon application of the external field, a behavior in contrast to other ionic materials such as LiBH4 or NaBH4. Since the electric field of the optical pulse reverses its direction every 1.3 fs, the electron is driven forth and back between the two sites with an extremely high speed of approximately one percent of the speed of light (c = 300.000 km/s).
|
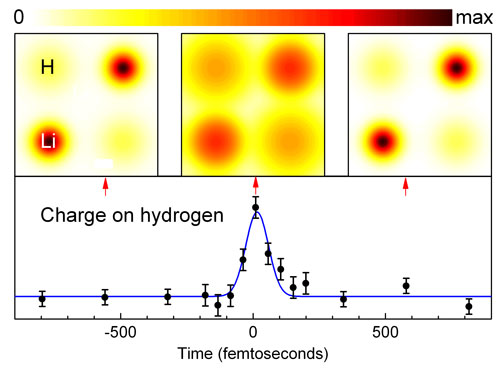 |
Fig. 2: Electron distribution of LiH in the grey shaded plane of Fig. 1 for times before (left panel), during (middle), and after (right) interaction of the LiH crystal with the strong electric field of the optical laser pulse. The contours show the electron density (charge per volume). The electric field moves electronic charge from the Li0.5+ to the H0.5- ion thereby making the material more ionic.
|
Immediately after the optical pulse the electrons return and the original electron distribution is restored. A qualitative explanation of the unexpected electron shift is as follows: The electric field accelerates the electrons in such a way that they are more uniformly distributed over the unit cell. Li has initially more electrons with the consequence of a loss of electrons during the optical pulse.
|
Because of the small electron number in LiH, all electrons contribute to this effect, making the electron distribution very sensitive to correlation effects. This picture is supported by theoretical calculations of the electron distribution. The manipulation of electron distributions by strong electric fields provides control over the material’s electric properties on an extremely short time scale, a fact that may lead to applications in ultrafast electrical switches.
|