One-dimensional (1D) materials
Nanomaterials are primarily categorized based on the dimensional characteristics they display. These dimensions are classified as zero-dimensional (0D), one-dimensional (1D), two-dimensional (2D), and three-dimensional (3D) nanomaterials, all of which fall within the nanoscale range.
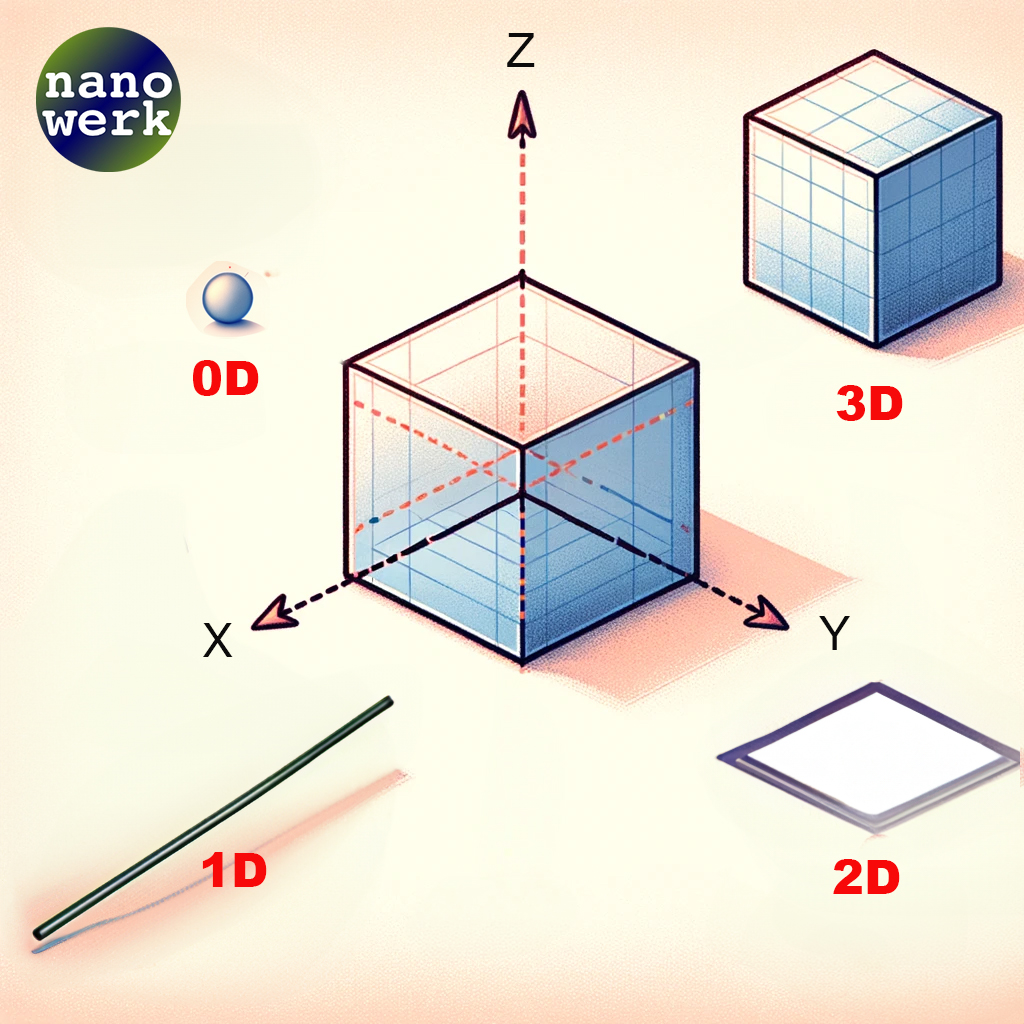
Classification of nanoscale dimensions. (© Nanowerk)
Unlike their zero-dimensional nanomaterials counterparts, which are confined in all three spatial dimensions, 1D nanomaterials are only confined in two, allowing for unique electrical, thermal, and mechanical properties.
One-dimensional nanomaterials offer a promising avenue for technological advancement. As researchers continue to unravel their potential, these nanoscale structures could be the key to breakthroughs in electronics, energy, healthcare, and more. By overcoming current challenges, the future of 1D nanomaterials shines bright, promising a new era of nanotechnology-driven solutions.
Alongside zero-dimensional (0D) and two-dimensional (2D) nanomaterials, one-dimensional nanostructures are transforming the nanoscale landscape. From redefining electronics to revolutionizing medicine, 1D nanomaterials possess seemingly limitless potential. As researchers overcome challenges and further explore their properties, these unique materials stand to create a future fueled by the innovative power of nanotechnology.
Understanding One-Dimensional Nanomaterials
One-dimensional nanomaterials stand out due to their unique structure, where their length significantly exceeds their width, confining them in two dimensions. This configuration results in quantized energy levels for precision control over electrical properties and an expansive surface-to-volume ratio, boosting reactivity and interactions with their environment.
While carbon nanotubes and semiconductor nanowires are among the most celebrated examples, their family extends far beyond, including metal nanowires, nanorods, and conductive polymers, each offering distinct advantages for various applications.
Metal Nanowires, such as silver and gold, are highly conductive and find applications in electronics and photonics. Their plasmonic properties are particularly valuable in sensors and imaging technologies, where they can enhance the detection of biomolecules or chemical species at incredibly low concentrations.
Nanorods, made from materials like zinc oxide, are utilized for their optical, electrical, and catalytic properties. In the field of renewable energy, zinc oxide nanorods are being explored for their ability to degrade organic pollutants under sunlight, offering a promising approach to environmental cleanup.
Conductive Polymers in one-dimensional form, such as polyaniline nanofibers, are revolutionizing flexible electronics and wearable technology. Their flexibility, combined with electrical conductivity, opens up new possibilities for smart textiles and flexible displays.
Synthesis Methods of One-Dimensional Nanomaterials: A Closer Look
The creation of one-dimensional nanomaterials involves sophisticated techniques that enable the precise control of their size, shape, and properties. While the vapor-liquid-solid (VLS) growth, template-assisted growth, and electrospinning methods are foundational, researchers are constantly refining these techniques and exploring new ones to meet the demands of specific applications.
Vapor-Liquid-Solid (VLS) Growth is a popular method for synthesizing semiconductor nanowires. It involves a catalytic metal nanoparticle that guides the one-dimensional growth of material from a vapor phase. The challenge here lies in controlling the diameter and orientation of the nanowires, which are crucial for their integration into electronic devices. Recent advancements have focused on manipulating the composition and size of the catalyst particles to achieve better control over the nanowire characteristics.
Template-Assisted Growth leverages nanoporous templates to guide the growth of nanomaterials into one-dimensional structures. This method is particularly versatile, allowing for the synthesis of nanotubes and nanowires from a wide range of materials, including metals, semiconductors, and polymers. However, the removal of the template without damaging the nanomaterial poses a significant challenge. Researchers are developing nondestructive techniques and biodegradable templates to circumvent this issue.
Electrospinning is a technique that produces polymer nanofibers and composite nanomaterials through the application of a high-voltage electric field. While electrospinning allows for the production of nanofibers with controllable diameters and compositions, achieving uniformity and alignment over large areas remains a challenge. Efforts to improve the collection methods and the use of auxiliary electric fields are underway to address these limitations.
In addition to these established methods, chemical vapor deposition (CVD) and laser ablation are gaining traction for their ability to produce high-quality nanomaterials. CVD, for instance, offers excellent control over the growth conditions, but scaling this process while maintaining material quality is challenging. Laser ablation provides a route to synthesize nanomaterials with minimal contaminants, though controlling the size distribution and yield requires further refinement.
Environmental Impact of One-Dimensional Nanomaterials
As the production and application of one-dimensional (1D) nanomaterials continue to expand, understanding and mitigating their environmental impact becomes increasingly important. The lifecycle of these nanomaterials—from synthesis to disposal—presents unique challenges and opportunities for environmental sustainability.
Synthesis and Production
The synthesis of 1D nanomaterials often requires high energy consumption and the use of hazardous chemicals, posing potential risks to both the environment and human health. For instance, the chemical vapor deposition (CVD) process, while effective for producing high-quality nanowires and nanotubes, typically operates at high temperatures and may involve toxic precursors. Similarly, the solvents and catalysts used in other synthesis methods can lead to the generation of harmful byproducts.
To reduce the environmental footprint of these processes, research is focusing on developing greener synthesis techniques. This includes the use of renewable energy sources, such as solar or wind power, to minimize energy consumption, and the exploration of bio-based precursors and solvents that are less toxic and more easily degradable. Additionally, recycling and reclaiming materials from nanowaste could significantly lower the demand for raw materials and reduce the generation of hazardous waste.
The applications of 1D nanomaterials in electronics, energy storage, and biomedical devices offer significant benefits, including enhanced efficiency and performance. However, the incorporation of nanomaterials into consumer products raises concerns regarding their lifecycle environmental impact. For example, nanomaterials embedded in electronic devices or clothing can enter the environment at the end of the product's life, potentially leading to accumulation in soil and water bodies.
Addressing these concerns requires the development of safe disposal and recycling methods for nanomaterial-containing products. Advances in nanomaterial recovery techniques, such as chemical treatment and physical separation, are critical for preventing nanowaste accumulation. Moreover, designing nanomaterials for environmental compatibility—such as biodegradable nanocomposites—can facilitate safer degradation and minimize ecological disruption.
Regulation and Management
The effective management of nanomaterials' environmental impact also depends on comprehensive regulatory frameworks. This involves not only the standardization of nanomaterial production and use but also the establishment of guidelines for disposal, recycling, and treatment of nanowaste. Regulatory bodies and researchers must work together to assess the risks associated with nanomaterials comprehensively, developing strategies that balance technological advancement with environmental stewardship.
Applications of One-Dimensional Nanomaterials
Electronics: Beyond Transistors and SensorsIn the realm of electronics, 1D nanomaterials like carbon nanotubes and semiconductor nanowires are pivotal in pushing the boundaries beyond traditional silicon-based technologies. Their exceptional electrical conductivity and unique quantum mechanical properties enable the development of ultrasmall, highly efficient electronic components.
For instance, carbon nanotubes are being used to create flexible, transparent conductive films for touchscreens and displays, offering superior performance over traditional indium tin oxide (ITO). Furthermore, semiconductor nanowires are integral in developing photodetectors and light-emitting diodes (LEDs) with enhanced efficiency and reduced energy consumption, paving the way for more sustainable electronic devices.
Energy: Enhancing Storage and Conversion
In the energy sector, 1D nanomaterials play a crucial role in addressing the global demand for clean, efficient, and sustainable energy solutions. High-performance batteries, such as lithium-ion batteries incorporating silicon nanowires as anodes, exhibit significantly increased charge capacity and cycle life compared to conventional batteries. This advancement is critical for both consumer electronics and electric vehicles, leading to longer battery life and reduced environmental impact.
Additionally, 1D nanomaterials are essential in the development of next-generation solar cells, including quantum dot solar cells and hybrid organic-inorganic perovskite solar cells, where they improve light absorption and charge transport, ultimately boosting efficiency and lowering costs.
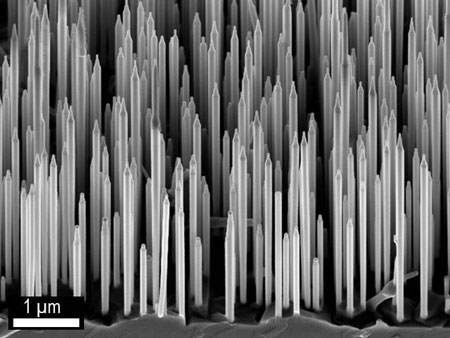
Tuning a single nanowire improves solar cells and LEDs. Electron microscope image of wurtzite GaA/AIGaAs core-shell nanowires. (Image: The Norwegian University of Science and Technology)
The medical field benefits immensely from the unique characteristics of 1D nanomaterials. Their ability to interact with biological systems at the molecular level makes them ideal for targeted drug delivery systems, where they can precisely deliver therapeutics to specific sites within the body, minimizing side effects and enhancing treatment efficacy. For example, nanotubes and nanowires can be functionalized with antibodies or ligands to target cancer cells, delivering chemotherapy agents directly to tumors while sparing healthy tissue. In diagnostics, 1D nanomaterials enhance the sensitivity and specificity of biosensors, enabling the early detection of diseases at the molecular level. This capability is crucial for timely intervention and improved patient outcomes.
Environmental and Agricultural Applications
Beyond these sectors, 1D nanomaterials are also making strides in environmental monitoring and agriculture. They are used in sensors capable of detecting pollutants and pathogens with high precision, contributing to cleaner water and air. In agriculture, nanoscale sensors and delivery systems improve crop health monitoring and the efficient use of fertilizers and pesticides, promoting sustainable farming practices.
Challenges and Future Prospects
While the potential of 1D nanomaterials is immense, challenges in scalability, integration, and environmental impact remain. The article would address these challenges, emphasizing ongoing research aimed at overcoming them. It would conclude with a perspective on the future of 1D nanomaterials, including the development of more sustainable synthesis methods, and the integration of these materials into complex systems, paving the way for innovations in technology and beyond.
Frequently Asked Questions about 1D Nanomaterials
What Are One-Dimensional Nanomaterials?
One-dimensional nanomaterials are nanostructures with one dimension typically below 100 nanometers. This unique form allows for exceptional electrical, thermal, and mechanical properties, making them ideal for various applications in electronics, energy, medicine, and more.
How Are One-Dimensional Nanomaterials Made?
They are synthesized using methods like chemical vapor deposition, electrospinning, and template-assisted growth, each technique tailored to produce nanotubes, nanowires, or nanorods with specific characteristics for their intended applications.
Why Are They Important for Electronics?
In electronics, 1D nanomaterials offer high electrical conductivity and unique quantum properties, enabling the development of next-generation transistors, sensors, and flexible conductive films, thereby enhancing device performance and energy efficiency.
Can One-Dimensional Nanomaterials Improve Energy Storage?
Yes, they play a crucial role in energy storage, particularly in batteries and supercapacitors, where they improve charge capacity, cycle life, and overall efficiency, driving advancements in both consumer electronics and electric vehicles.
How Do They Benefit the Medical Field?
One-dimensional nanomaterials are used in targeted drug delivery systems and biosensors, offering precise treatment options with minimal side effects and enabling early detection of diseases, thus improving patient outcomes.
What Challenges Are Associated with 1D Nanomaterials?
Challenges include controlling their synthesis for specific applications, understanding their long-term environmental and health impacts, and addressing concerns regarding nanowaste management and potential toxicity.
Are They Environmentally Friendly?
The environmental impact of 1D nanomaterials varies. While they offer sustainable solutions in energy and electronics, concerns about nanowaste and the lifecycle assessment of these materials highlight the need for responsible manufacturing and disposal practices.
How Are They Integrated into Consumer Products?
They are integrated into consumer products like electronics, clothing, and cosmetics, enhancing product performance through improved electrical properties, durability, and added functionalities like antimicrobial properties.
What Future Applications Can We Expect?
Future applications include more efficient solar cells, smart textiles, advanced drug delivery systems, and nanoscale devices for computing and data storage, potentially revolutionizing multiple industries with their innovative properties.