Bose-Einstein Condensate: The Fifth State of Matter
What is a Bose-Einstein Condensate?
A Bose-Einstein condensate (BEC) is an exotic state of matter that forms when a dilute gas of bosonic particles is cooled to temperatures very close to absolute zero. At these extremely low temperatures, a large fraction of the bosons occupy the lowest quantum state, forming a coherent superfluid. BECs exhibit unique quantum properties on a macroscopic scale, making them a fascinating subject of study in the fields of atomic physics, quantum optics, and condensed matter physics.
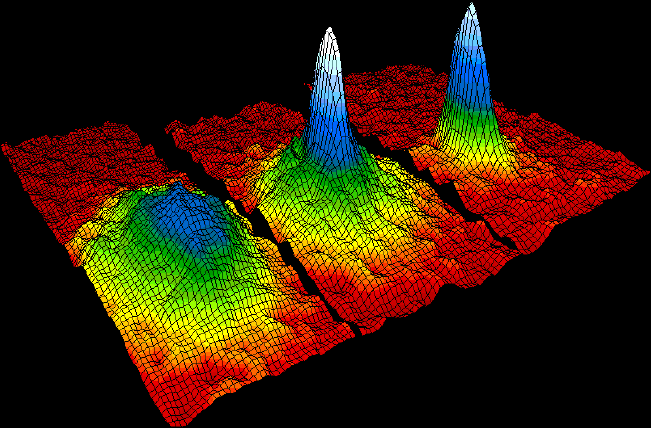
Theoretical Foundations
The concept of Bose-Einstein condensation was first predicted by Satyendra Nath Bose and Albert Einstein in 1924. They showed that a gas of non-interacting bosons (particles with integer spin) would condense into the lowest energy state at sufficiently low temperatures. This condensation occurs because bosons, unlike fermions, do not obey the Pauli exclusion principle and can occupy the same quantum state.
The critical temperature for Bose-Einstein condensation depends on the density of the gas and the mass of the bosonic particles.
Experimental Realization
The first experimental realization of Bose-Einstein condensation was achieved in 1995 by Eric Cornell, Carl Wieman, and Wolfgang Ketterle, who were awarded the Nobel Prize in Physics in 2001 for their pioneering work. They created BECs using ultracold atomic gases, such as rubidium and sodium, cooled to temperatures on the order of 100 nanokelvin using a combination of laser cooling and evaporative cooling techniques.
Since then, Bose-Einstein condensation has been observed in various atomic species, as well as in molecules, quasiparticles (such as exciton-polaritons and magnons), and even photons in a cavity.
Properties and Applications
Bose-Einstein condensates exhibit remarkable properties that make them an ideal platform for studying quantum phenomena and developing novel technologies:
Superfluidity
BECs behave as superfluids, meaning they can flow without friction. This property arises from the coherent nature of the condensate, where all particles occupy the same quantum state and move collectively. Superfluidity in BECs has been demonstrated through the observation of quantized vortices and the absence of viscosity.
Atom Interferometry
The coherence and wave-like nature of BECs make them suitable for atom interferometry, a technique that uses the wave properties of atoms to measure various physical quantities with unprecedented precision. BEC-based atom interferometers have been used for gravitational wave detection, precision measurements of fundamental constants, and inertial sensing.
Quantum Simulation
BECs provide a versatile platform for simulating complex quantum systems, such as solid-state materials, superconductors, and even black holes. By manipulating the interactions between the atoms in a BEC, researchers can engineer artificial quantum systems that mimic the behavior of real materials, enabling the study of quantum phenomena that are difficult to observe directly.
Quantum Information Processing
The coherent and entangled nature of BECs makes them a promising candidate for quantum information processing. BECs can be used to create quantum bits (qubits) and perform quantum logic operations, paving the way for the development of quantum computers and quantum communication networks.
Challenges and Future Directions
Despite the significant progress in BEC research, several challenges remain. One of the main challenges is the limited lifetime of BECs due to various loss mechanisms, such as three-body recombination and atom-atom interactions. Extending the lifetime of BECs is crucial for their practical applications in quantum technologies.
Future research directions in BEC physics include the exploration of novel cooling and trapping techniques, the study of strongly interacting and dipolar BECs, and the integration of BECs with other quantum systems, such as superconducting circuits and optomechanical resonators. These advancements will further expand the potential applications of BECs in quantum sensing, simulation, and information processing.
Further Reading
Frontiers in Physics, Evolution of Bose–Einstein condensate systems beyond the Gross–Pitaevskii equation
Nature Reviews Physics, Non-equilibrium Bose–Einstein condensation in photonic systems