Superconducting Quantum Interference Device (SQUID): Ultrasensitive Magnetic Field Detection
What is a SQUID?
A Superconducting Quantum Interference Device (SQUID) is an extremely sensitive magnetometer that measures very subtle magnetic fields. It exploits the quantum mechanical properties of superconductivity and Josephson junctions to detect magnetic fields as small as 5 aT (5×10-18 T). SQUIDs are the most sensitive magnetometers currently available and have found applications in various fields, including biomedical imaging, geophysical exploration, and quantum computing.
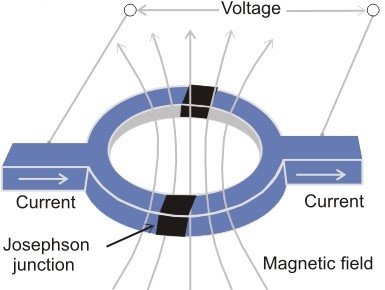
Types of SQUIDs
There are two main types of SQUIDs: DC SQUIDs and RF SQUIDs.
- DC SQUID: A DC SQUID consists of two Josephson junctions connected in parallel in a superconducting loop. When an external magnetic field is applied, it induces a current in the loop, which is detected by measuring the voltage across the junctions. DC SQUIDs are more commonly used due to their higher sensitivity and better noise performance.
- RF SQUID: An RF SQUID has a single Josephson junction in a superconducting loop, coupled to a radio-frequency (RF) circuit. The external magnetic field modulates the inductance of the loop, which is detected by monitoring the resonant frequency of the RF circuit. RF SQUIDs are simpler to fabricate but have lower sensitivity compared to DC SQUIDs.
Working Principle of SQUIDs
The working principle of SQUIDs relies on two quantum mechanical phenomena: flux quantization and Josephson tunneling.
Flux Quantization
In a superconducting loop, the magnetic flux is quantized in units of the magnetic flux quantum, Φ0 = h/2e ≈ 2.07 × 10-15 Wb, where h is Planck's constant and e is the electron charge. This means that the magnetic flux threading the loop can only take discrete values that are integer multiples of Φ0.
Josephson Tunneling
A Josephson junction consists of two superconductors separated by a thin insulating barrier. Cooper pairs (pairs of electrons) can tunnel through the barrier without any voltage applied, giving rise to a supercurrent. The maximum supercurrent that can flow through the junction, called the critical current (Ic), is highly sensitive to the magnetic flux threading the SQUID loop.
In a DC SQUID, the external magnetic field induces a current in the superconducting loop, which is added to or subtracted from the bias current flowing through the Josephson junctions. This results in a periodic modulation of the voltage across the junctions as a function of the applied magnetic flux, with a period of Φ0. By measuring this voltage modulation, the external magnetic field can be precisely determined.
Advantages of SQUIDs
SQUIDs offer several advantages over other magnetometers:
- Ultrahigh Sensitivity: SQUIDs can detect magnetic fields as small as 5 aT, making them the most sensitive magnetometers available. This exceptional sensitivity enables the detection of extremely weak magnetic signals, such as those generated by the human brain or heart.
- Wide Bandwidth: SQUIDs have a wide frequency response, allowing them to measure magnetic fields from DC to several GHz. This broad bandwidth is crucial for applications that require the detection of fast-changing magnetic signals, such as in magnetoencephalography (MEG).
- Low Noise: SQUIDs have very low intrinsic noise, which enables the detection of small magnetic signals that would otherwise be obscured by noise. The low noise performance of SQUIDs is particularly important for applications that require high signal-to-noise ratios, such as in quantum computing and precision measurements.
Applications of SQUIDs
SQUIDs have found numerous applications across various fields, leveraging their ultrahigh sensitivity and wide bandwidth:
Biomedical Imaging
SQUIDs are used in magnetoencephalography (MEG) and magnetocardiography (MCG) to measure the weak magnetic fields generated by the brain and heart, respectively. These techniques provide valuable information about the function and health of these organs, aiding in the diagnosis and treatment of neurological and cardiac disorders.
Geophysical Exploration
SQUIDs are employed in geophysical surveys to detect the magnetic signatures of mineral deposits, oil and gas reservoirs, and underground water sources. The high sensitivity of SQUIDs allows for the detection of deep and subtle magnetic anomalies, aiding in the exploration and mapping of Earth's subsurface.
Quantum Computing
SQUIDs are used as the building blocks of superconducting qubits, the fundamental units of quantum information in superconducting quantum computers. The sensitivity and low noise of SQUIDs enable the precise control and readout of the quantum states of the qubits, which is essential for performing quantum computations.
Challenges and Future Perspectives
Despite the remarkable capabilities of SQUIDs, several challenges need to be addressed for their widespread adoption. One of the main challenges is the requirement for cryogenic cooling to maintain the superconducting state of the SQUID. This necessitates the use of complex and expensive cooling systems, limiting the portability and accessibility of SQUID-based devices.
Future research in SQUID technology will focus on the development of high-temperature superconducting (HTS) SQUIDs, which can operate at liquid nitrogen temperatures (77 K) instead of liquid helium temperatures (4.2 K). HTS SQUIDs would significantly reduce the cooling requirements and enable more compact and cost-effective SQUID systems. Additionally, the integration of SQUIDs with other technologies, such as atomic magnetometers and optically pumped magnetometers, will be explored to combine the strengths of different magnetometer technologies for advanced applications.
Further Reading
Nature Physics, Superconducting quantum interference at the atomic scale