Superwettability: Controlling Surface Interactions at the Nanoscale
What is Superwettability?
Superwettability refers to the extreme wetting properties of a surface, characterized by its ability to interact with liquids in a highly controlled manner. Superwettable surfaces can exhibit either superhydrophobicity (extreme water repellency) or superhydrophilicity (extreme water attraction), depending on their chemical composition and physical structure at the nanoscale.
The degree of wettability is determined by measuring the contact angle between a liquid droplet and the surface. A contact angle greater than 150° indicates superhydrophobicity, while a contact angle close to 0° indicates superhydrophilicity. These unique properties have garnered significant attention in various fields, including self-cleaning surfaces, anti-fouling coatings, and microfluidic devices.
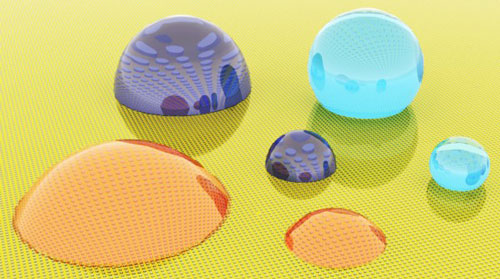
Superhydrophobicity
Superhydrophobic surfaces exhibit extreme water repellency, with water contact angles greater than 150°. This phenomenon is inspired by nature, with examples such as the lotus leaf and butterfly wings. The key to achieving superhydrophobicity lies in the combination of low surface energy materials and hierarchical micro/nanostructures on the surface. These structures trap air pockets, creating a composite interface that minimizes contact between the liquid and the solid surface, resulting in high contact angles and low adhesion. Materials like Teflon (polytetrafluoroethylene) and silicone rubber are commonly used to create superhydrophobic surfaces due to their low surface energy.
Applications of Superhydrophobic Surfaces
- Self-Cleaning Surfaces: Superhydrophobic surfaces can easily shed water droplets, carrying away dirt and contaminants, making them ideal for self-cleaning applications in buildings, solar panels, and textiles.
- Anti-Icing Coatings: The water-repellent nature of superhydrophobic surfaces can prevent the accumulation of ice, making them suitable for anti-icing applications in aviation, power transmission, and refrigeration systems.
- Oil-Water Separation: Superhydrophobic and superoleophilic surfaces can selectively absorb oil while repelling water, enabling efficient oil-water separation for environmental remediation and industrial wastewater treatment.
Superhydrophilicity
Superhydrophilic surfaces exhibit extreme water attraction, with water contact angles close to 0°. These surfaces are characterized by their high surface energy and the presence of hydrophilic functional groups. When a water droplet comes in contact with a superhydrophilic surface, it spreads out completely, forming a thin film. Materials like titanium dioxide (TiO2) and silicon dioxide (SiO2) are known for their superhydrophilic properties, particularly when exposed to UV light, which induces photocatalytic reactions and increases surface hydrophilicity. This property is exploited in various applications, such as anti-fogging coatings, self-cleaning windows, and biomedical devices.
Applications of Superhydrophilic Surfaces
- Anti-Fogging Coatings: Superhydrophilic coatings prevent the formation of water droplets on surfaces, eliminating fogging and maintaining clear visibility. This is particularly useful in automotive windshields, goggles, and camera lenses.
- Self-Cleaning Windows: Superhydrophilic coatings on windows allow water to spread out and form a thin film, which can easily wash away dirt and contaminants, maintaining a clean and transparent surface.
- Biomedical Devices: Superhydrophilic surfaces can enhance the biocompatibility and anti-fouling properties of medical implants and devices, reducing the risk of infection and improving patient outcomes.
Fabrication Methods
Various fabrication methods have been developed to create superwettable surfaces, including:
- Chemical Etching: Chemical etching involves selectively removing material from a surface to create micro/nanostructures. This can be achieved through wet etching using acids or bases, or dry etching using plasma or reactive ion etching.
- Layer-by-Layer Deposition: Layer-by-layer deposition involves the sequential adsorption of oppositely charged materials onto a substrate, allowing precise control over the surface composition and thickness.
- Sol-Gel Processing: Sol-gel processing involves the hydrolysis and condensation of metal alkoxide precursors to form a network of metal oxide nanoparticles. This method enables the creation of porous and hierarchical structures with tunable wettability.
- Electrospinning: Electrospinning is a versatile technique that uses an electric field to draw charged polymer solutions into ultrafine fibers. By controlling the composition and morphology of the electrospun fibers, superwettable surfaces can be fabricated.
Challenges and Future Perspectives
Despite the significant progress in superwettable surface research, several challenges remain. One of the main challenges is the long-term durability and mechanical stability of superwettable surfaces, particularly in harsh environments. The development of robust and scalable fabrication methods is crucial for the widespread adoption of superwettable surfaces in practical applications.
Future research will focus on the design of stimuli-responsive superwettable surfaces that can switch between different wetting states in response to external triggers, such as temperature, pH, or light. The integration of superwettable surfaces with other functional materials, such as sensors, actuators, and energy harvesting devices, will open up new opportunities for smart and multifunctional surfaces. Additionally, the exploration of superwettable surfaces for biomedical applications, such as drug delivery and tissue engineering, will be a key area of investigation.
Further Reading
Angewandte Chemie International Edition, Bioinspired Super-Wettability from Fundamental Research to Practical Applications
European Polymer Journal, Recent advances in superhydrophobic surfaces for practical applications: A review