Oct 28, 2020 |
Direct observation of a single electron's butterfly-shaped distribution in titanium oxide
(Nanowerk News) The functions and physical properties of solid materials, such as magnetic order and unconventional superconductivity, are greatly influenced by the orbital state of the outermost electrons (valence electrons) of the constituent atoms.
|
In other words, it could be said that the minimal unit that determines a solid material's physical properties consists of the orbitals occupied by the valence electrons.
|
Moreover, an orbital can also be considered a minimal unit of "shape," so the orbital state in a solid can be deduced from observing the spatially anisotropic distribution of electrons (in other words, from how the electron distribution deviates from spherical symmetry).
|
The orbital states in elements are basic knowledge that can be found in quantum mechanics or quantum chemistry textbooks. For example, it is known that the 3d electrons in transition elements such as iron and nickel have characteristic butterfly-type or gourd-type shapes (see Fig. 1(a)).
|
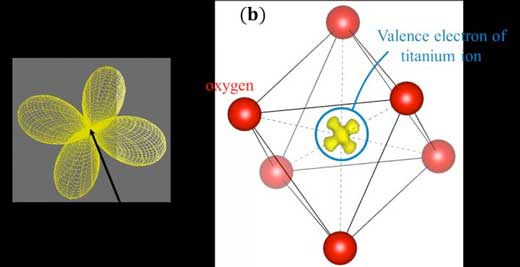 |
Figure 1. (a) Distribution of a butterfly-shaped 3d electron orbital. (b) Valence electron density distribution around the titanium (Ti3+) ion at the centre of the titanium oxide (TiO6) octahedron obtained by the CDFS analysis developed by the research team for this project. (Image: Shunsuke Kitou)
|
However, until now, it has been extremely difficult to observe the real-space distribution of such electron orbitals directly.
|
Now, a research collaboration between Nagoya University, University of Wisconsin-Milwaukee, Japan's RIKEN and Institute for Molecular Science, the University of Tokyo, and the Japan Synchrotron Radiation Research Institute (JASRI), has observed the spatial distribution of a single valence electron at the centre of an octahedron-shaped titanium oxide molecule, using synchrotron X-ray diffraction (see Fig. 1(b)) (Physical Review Research, "Collapse of the simple localized 3d1 orbital picture in Mott insulator").
|
To analyse the X-ray diffraction data from the titanium oxide sample, the team developed a Fourier synthesis method in which data from each titanium ion's inner shell electrons - which do not contribute to the compound's physical properties - are subtracted from the total electron distribution of each ion, leaving only the butterfly-shaped valence electron density distribution. The method is called core differential Fourier synthesis (CDFS).
|
Furthermore, a closer look at the butterfly-shaped electron density revealed that high density remained in the central region (see Fig. 2(a)), in contrast with bare titanium in which electrons do not exist at the centre because of the node of the 3d orbital (see Fig. 1(a)).
|
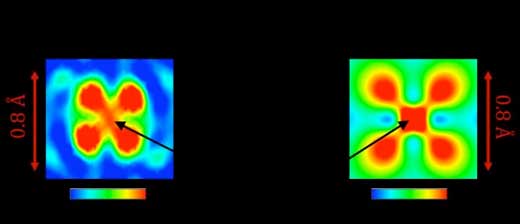 |
Figure 2. Cross-sectional view of the valence electron density distribution of Ti3+ ion obtained by (a) the CDFS analysis and (b) the first-principles calculation. (Image: Shunsuke Kitou)
|
After careful data analysis, it was found that the electron density at the centre consists of the valence electrons occupying the hybridized orbital generated by the bond between titanium and oxygen. First-principles calculations confirmed this non-trivial orbital picture and reproduced the results of the CDFS analysis very well (see Fig. 2(b)). The image directly demonstrates the well-known Kugel-Khomskii model of the relationship between the magnetic and orbital-ordered states.
|
The CDFS method can determine the orbital states in materials regardless of the physical properties and can be applied to almost all elements and without the need for difficult experiments or analytical techniques: the method requires neither quantum-mechanical nor informatic models, so bias introduced by analysts is minimized.
|
The results may signal a breakthrough in the study of orbital states in materials. The CDFS analysis will provide a touchstone for a complete description of the electronic state by first-principles or other theoretical calculations.
|