Nov 09, 2011 |
Self-assembling biomimetic nanostructures
|
(Nanowerk News) The design of biological molecules that mimic living structures and tissue is emerging as one of the most valuable strategies in bioengineering. Such biomimetic molecules, coupled with increasingly accurate simulations of cellular growth and responses, are leading to a wide range of regenerative applications as well as the development of controlled drug delivery systems and biochips for pharmaceutical research and diagnosis. In a significant step forward in the field, Charlotte Hauser and co-workers at the A*STAR Institute of Bioengineering and Nanotechnology (IBN) have designed a new class of ultrasmall peptides capable of self-assembling into a variety of structures such as membranes, micelles, tubules and gels that are suitable for application in tissue engineering and regenerative medicine.
|
Bioengineering breakthrough
|
The unique class of self-assembling peptides designed by the IBN research team consists of only 3 to 7 amino acids, in contrast to conventional peptides that usually require 16 to 32 amino acids. Each peptide molecule is characterized by a water-soluble 'polar head' and a water-insoluble 'tail', and this ampiphilic property enables the molecules to self-assemble spontaneously in water to form hydrogels—stiff, water-based gels held together by stable fibrous structures. These natural peptide-based hydrogels offer an attractive, low-cost alternative for the manufacture of biomimetic materials, as they do not require the addition of enzymes or chemical agents during the process of formation.
|
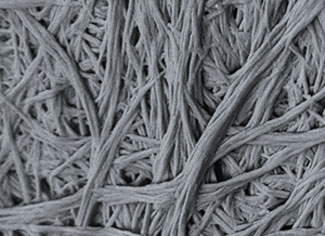 |
Scanning electron microscopy image of fibrous structures formed by self-assembly of ultrasmall peptides. These structures closely resemble collagen fibers. (© 2011 PNAS)
|
Using field-emission scanning electron microscopy (FESEM), the researchers observed that the structure of the peptide-derived hydrogels is remarkably similar to collagen, the most abundant protein in mammals and the main component of connective tissue found in tendons, cartilage, ligaments, bone, adipose tissue and lymphatic tissue.
|
New treatments for spinal disc damage
|
The biocompatibility of the peptide-derived hydrogels developed by Hauser and her co-workers have particularly promising applications in the development of new treatment options for cartilage repair and spinal disc replacement. "We are currently investigating the potential of our peptide scaffold for the treatment of degenerative disc disease," says Hauser. "We hope to offer an injection therapy that would render invasive surgical treatment obsolete, to meet the clinical need for disc prostheses that could inhibit or repair early-stage disc damage."
|
Degenerative disc disease—a debilitating condition caused by the erosion of a collagenous substance called nucleus pulposus in the middle of the spinal disc—currently affects approximately 85% of people by the age of 50. New curative therapies afforded by next-generation bioengineering are therefore of particular interest in orthopedics. Existing treatments often involve implanting an artificial disc made of metal or rigid plastic, but the design of biocompatible hydrogels that are capable of absorbing mechanical shock and providing flexibility by closely replicating the properties of a natural spinal disc could prove to be a much more effective treatment while at the same time being much less invasive.
|
 |
Scanning electron microscopy image of the honeycomb-like structure of the peptide scaffolds, which enable the hydrogels to contain large amounts of water. (© Nano Today)
|
Hauser and her co-workers discovered that their peptide-derived hydrogels are heat-resistant up to 90°C and demonstrate tunable, high mechanical strength. The stiffness of the hydrogels can also be tailored by altering the concentration of the peptide, besides changing the ionic strength and pH. The entrapment of large amounts of water, in some cases up to 99.9%, results in the formation of three-dimensional peptide scaffolds that resemble a porous honeycomb-like structure. The tunable mechanical properties of the hydrogels may lead to the development of biomaterials that could be more readily customized to individual patients.
|
Insights into degenerative diseases
|
In another study, Hauser and her co-workers discovered that the self-assembly of ultrasmall peptides mimics the formation of amyloids, a common pathological feature in degenerative diseases such as Alzheimer's, Parkinson's and prion diseases, as well as type II diabetes.
|
The ultrasmall peptides could therefore serve as a model system to address fundamental questions about disease onset and progression. Further investigations into the self-assembly process could also lead to the development of new therapeutic approaches specifically targeted at the prevention or control of amyloid formation.
|
Diverse applications
|
The IBN's research into biomimetics is not restricted to therapeutic applications. Recent developments at the institute include the design of coatings for bioartificial kidneys and the synthesis of new antimicrobial agents.
|
As the new class of ultrasmall peptides designed by Hauser's team consists of simple, aliphatic amino acids similar to those that existed in the 'primordial soup' from which all life is thought to have evolved, studying how these molecules interact and assemble spontaneously could provide important clues to the origin of life and the mechanisms underlying evolution.
|
By altering the amino acid sequence of the ultrasmall peptides, Hauser and her co-workers have discovered that it is possible to form a surprisingly wide variety of nanostructures, including hollow nanospheres, short, flat fibers, elongated helical fibers and spiderweb-like structures. The shape and stability of the nanostructures is critically dependent on factors including the length of the hydrophobic tail and the polar nature of the head of the peptide, as well as the pH and peptide concentration used in the preparation of the nanostructure. The ability to tune the physical and chemical properties of peptide nanostructures may also make them suitable for applications in novel biosensing devices.
|
The most exciting possibilities for self-assembling peptides, however, are in biomedical applications due to their close resemblance to natural polypeptides. Hauser envisions that new advances in wound healing and skin treatment, as well as cosmetic applications, may be available within the next 5 or 7 years. To realize this goal, Hauser and her co-workers are currently focusing on clinical trials and establishing industrial partnerships. "IBN aims to create new biomaterial platforms based on nanotechnology," says Jackie Y. Ying, IBN's executive director. "This unique class of ultrasmall peptides are biomimetic, and have excellent potential as cell culture substrates and tissue engineering scaffolds."
|