Jun 15, 2021 |
Plant biologists solve major cell puzzle on path to leaf engineering
|
(Nanowerk News) Leaves are the primary plant organs responsible for photosynthesis. Their size, shape and angles — all affected by cell patterning and growth — can also expose more of their surface to the sun, increasing energy stores and grain production in crops. Epidermal cells on the outer leaf surface control the growth of the organ and form in highly convoluted jigsaw-like shapes. Understanding how plant cells control those complex cell sizes and shapes is a major goal of plant biology.
|
In a computational modeling and cell biology paper in the journal Nature Plants ("Real-time conversion of tissue-scale mechanical forces into an interdigitated growth pattern"), Purdue’s Dan Szymanski, a professor in the Department of Botany and Plant Pathology, discovered that tensile force patterns in thin, pressurized cell walls carry information that pattern cell and tissue morphogenesis. The findings uncover a conserved cellular basis of plant growth and are key to understanding leaf architecture and potentially modifying it in the future to improve plants.
|
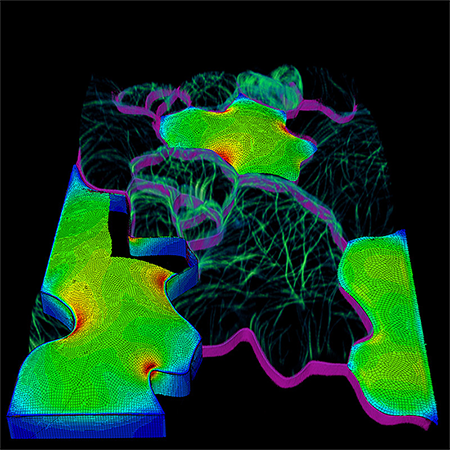 |
This patch of leaf epidermal tissue shows a mosaic of living and computationally modeled cells. The image data of the living cells were obtained using plasma membrane (magenta) and microtubule (green) marker proteins. The finite element mechanical models of the cells show tensile force patterns. (Image: Dan Szymanski)
|
“The leaf epidermis is like an exoskeleton, the growth of which determines the size and shape of organs,” Szymanski said. “We’re showing how groups of cells can function as dynamic building blocks for the organ. The tensile force patterns in the cell wall are decoded by a cytoskeleton-cell wall system that determines how cells in the tissue interact and grow at maximal rates. These cellular growth patterns can be maintained for days to affect tissue and organ-level morphology.”
|
Szymanski, in collaboration with Joseph Turner, a professor of mechanical and materials engineering at the University of Nebraska-Lincoln, showed that cells have areas of high tensile force in their walls. The magnitude and direction of these forces are sensed and mirrored by the microtubule cytoskeleton.
|
Microtubules, hollow protein-based tubes, pattern the synthesis of cellulose fibers in the cell wall. The extracellular fibers accumulate and orient in these high-pressure areas to create patterns of cell expansion that maintain tissue integrity and enable the rapid expansion of thin-bladed leaves.
|
A lobed morphology in internal cell types in the leaf enables efficient gas exchange for photosynthesis, and this cellular trait affects yield in major crop species. Szymanski’s group discovered a general mechanism for lobe formation in any plant cell type.
|
Realistic mechanical computational models of the cell wall showed that the microtubule system mirrors the magnitude and direction of wall tensile forces. Szymanski said.
|
“We quantitatively showed that forces in the wall are sensed continuously by the microtubule system, he said.
|
These microtubules, which were analyzed in real time by Samy Belteton, a graduate student in Szymanski’s lab, using live cell imaging, pattern the movement of cellulose synthase machines that make extracellular fibers that locally reinforce cell wall domains. This enables the tissue to grow adaptively in the expanding leaf.
|
Szymanski and his collaborators identified the location and magnitude of the important tensile forces and the identity of the subset of microtubules controlling cell shape for the first time. This work provides important insights into how mechanical signaling at the cell periphery occurs and how it dictates local growth behaviors.
|
Szymanski’s group will continue to determine how these cellular scale events operate at the organ level to define leaf traits. Understanding the how these cellular building blocks of leaves acquire their sizes and shapes provides a basis to engineer leaf architectures that are optimal for crop productivity.
|