Posted: Jul 03, 2015 |
A 'movie' of ultrafast rotating molecules at a hundred billion per second
|
(Nanowerk News) Can you imagine how subnano-scale molecules make an ultrafast rotation at a hundred billion per second? Do the ultrafast rotating subnano-scale molecules show a wave-like nature rather than particle-like behavior? The Japanese research team led by Professor Yasuhiro Ohshima at the Tokyo Institute of Technology, and Dr Kenta Mizuse at the Institute for Molecular Science, National Institutes of Natural Sciences, successfully took sequential "snapshots" of ultrafast unidirectionally rotating molecules at a hundred billion per second.
|
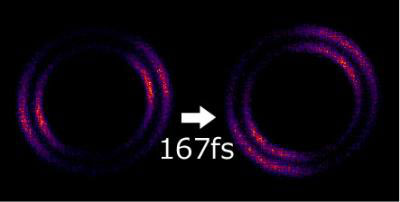 |
"Snapshots" of ultrafast rotating nitrogen molecules at a hundred billion per second (femtosecond = a quadrillionth part of one second). (Image: IMS/NINS)
|
To visualize such an ultrafast molecular rotation, the team developed a Coulomb explosion imaging setup with regulating rotational direction by a pair of time-delayed, polarization-skewed laser pulses. In the sequential "snapshots", the team successfully reported high-resolution direct imaging of direction-controlled rotational wave packets (RWPs) in nitrogen molecules, and the quantum wave-like nature was successfully observed. The result will guide more sophisticated molecular manipulations, such as an ultrafast molecular "stopwatch". This result is published in Science Advances ("Quantum unidirectional rotation directly imaged with molecules").
|
Rotational wave packets (RWPs) are time-varying states of motion of rotating microscopic objects like molecules, and they change shapes in an ultrafast time scale, typically some parts in a trillion second. More importantly, because RWPs are governed by the fundamental microscopic physical laws, quantum mechanics, they show a wave-like nature, much different from what macroscopic things exhibit. So, RWPs are one of the ideal play grounds for examining the connection between quantum and classical worlds.
|
In the present study, the RWPs were created by using a pair of ultrafast laser pulses, of which mutual delay and polarization were properly adjusted. In addition, by using a specially designed ion-imaging setup, the team got images of unidirectional RWPs at a viewing angle that the previous 2D imaging studies could not adopt. As a result, the team succeeded in recording a series of images of time-varying molecular angular distribution with high-spatial resolution, which is nothing but a "movie" on the RWPs with a defined sense of rotation. The movie clearly shows the wave-like nature of the RWPs. Multiple running waves get together at some time to give a highly concentrated spatial orientation and split after a while into parts having different angular velocities, while the overall movement keeps rotating in one direction. This propagation of wave packets may well be expected as a pedantic example of a simple quantum system like rotating molecules in free space, for which mutual interaction is essentially inoperative. Nevertheless, it has never been visualized experimentally so far.
|
There have been many proposals for novel application of unidirectional RWPs. For instance, unidirectionally rotating molecular gas ensembles are expected to make sophisticated polarization shaping of ultrafast light pulses. In addition, unidirectional RWPs exhibiting cogwheel like motion are expected to be used as a "stopwatch" to measure the precise time difference between pulses from two independent ultrafast laser systems.
|
In a purely fundamental point of view, on the other hand, it is so challenging to experimentalists to explore how the wave-like nature of RWPs is approaching the particle-like behavior for a macroscopic object. It is also of great interest to experimentally track the loss of the wave-like character by a mutual or external interaction. Professor Ohshima said, "We hope our high-resolution RWP imaging to be applicable in making a movie on such crossovers from quantum to classical worlds."
|