Apr 02, 2014 |
Don't gamble, use physics for LED lighting
|
(Nanowerk News) Targeting a desired color for a white light-emitting diode is like spraying a savannah with buckshot in the hope of fortuitously downing a well-hidden deer. Optical engineers collect large amounts of data and perform numerous Monte Carlo simulations to hit upon the right mixture of luminescent phosphor and scatterers for a white LED, one with color characteristics that customers want for their illumination applications.
|
Scientists at the University of Twente and Philips have reached an understanding of the transport of light in white LEDs in physical terms. It turns out that light scattering can be well understood as a random walk of a drunken sailor with a step size called the mean free path. Moreover, the absorption of blue light is understood as the endpoint of the random walk. Dr. Vanessa Leung and colleagues use this information to propose new design rules in terms of the size step size relative to the thickness of the phosphor layer. They relate the interplay of random walks leading to scattering and those leading to absorption to co-ordinates on a color diagram.
|
This approach provides a physics-driven guide to designing white LEDs, reducing the amount of trial-and-error involved to obtain the optimal color. Their results appear in the leading journal Optics Express that is published by the Optical Society of America ("Interplay between multiple scattering, emission, and absorption of light in the phosphor of a white light-emitting diode").
|
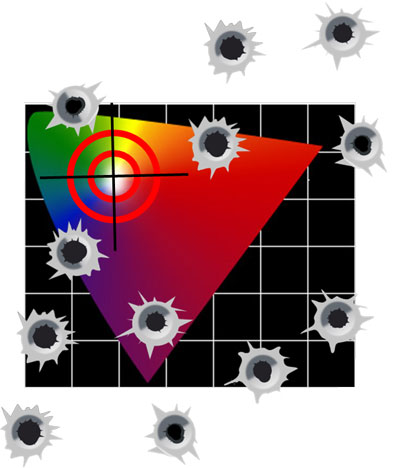 |
Figure 1: Cartoon on how to hit the optimal color point (see crosshair) of a white LED guided by the physics of diffusion vs. trial-and-error or “buckshot”. The physics approach is “greener” in the sense that precious resources will be saved.
|
Numerical calculations for white LEDs are currently not only based on the independent solution of specific equations, but require many additional “free adjustable parameters” in order to be effective. Determining these parameters is time-consuming and costly for the lighting designer who needs a simple, intuitive understanding of the effect of a minor change to a material or optical property.
|
The combination of diffusive light scattering and energy conversion in phosphor-converted LEDs is most often described by ray-tracing and Monte Carlo techniques. These numerical techniques seek to reproduce the potential configurations of light in a complex system by describing the transport behavior on a microscopic level. A large number of calculated possibilities are accumulated to produce an averaged outcome.
|
Dr. Leung explains, “As such, these methods are not designed to give in one calculation an ensemble-averaged view of light transport based on a few simple input parameters. The predictive power offered by an analytical, macroscopic approach could guide the evolution of efficient phosphor layers with improved optical properties.”
|
In addition, simulations can be computationally demanding and time-consuming, although recent efforts in parallel processing such as GPU programming offer promising speedups. In the application area of white LEDs, numerical approaches still require a significant input of measured data in order to predict relevant optical parameters correctly.
|
An improved description of multiple light scattering can be obtained by analytical theories originating from nanophotonics, wherein multiple scattering of light is described from first principles. The advantage of such ab initio models over ray tracing is that one obtains fundamental physical insight, starting from the detailed nanostructure of a sample. Moreover, calculating optical properties from a model is computationally much faster than performing a ray tracing simulation.
|
 |
Figure 2: Top: The spectrum of a typical white LED. Bottom: Total relative intensity, a measurement equal to absorption, emission, and scattering for a phosphor density of 4 wt% YAG:Ce3+ (green circles). The total transmission is identified to be a measurement of absorption and scattering only (red diamonds). Below wavelength there is no emission and above a wavelength a high-pass filter was used to eliminate the emission contribution.
|
The team measured the broadband diffuse transmission through phosphor plates of varying YAG:Ce3+ density. They distinguished the spectral ranges where absorption, scattering, and re-emission dominate (Figure 2). Using diffusion theory, they derived the transport and absorption mean free paths from first principles. It was found that both transport and absorption mean free paths are on the order of the plate thickness. This means that phosphors in commercial LEDs operate well within an intriguing albedo range around 0.7. The authors discuss how salient parameters that can be derived from first principles control the optical properties of a white LED (Figure 3).
|
The Team: the research was performed by Dr. Vanessa Leung, Prof. Dr. Ad Lagendijk, Prof. Dr. Allard Mosk, and Prof. Dr. Willem Vos from the Complex Photonics Systems (COPS) Chair at the MESA+ Institute for Nanotechnology of the University of Twente in Enschede, The Netherlands, and by Dr. Teus Tukker at Philips Research and by Dr. Wilbert IJzerman at Philips Lighting in Eindhoven, The Netherlands.
|
 |
Figure 3: Color coordinates of the phosphor plates with increasing phosphor density on the CIE 1960 (u', v') chromaticity diagram (filled red circles). The plates were illuminated by a reference light source with u' = 0.21, v' = 0.30 (open red circle). The solid black line denotes the boundary of the (u', v’) color space. The dashed blue line denotes the Planckian locus. Combining phosphor plates of increasing density with the reference light source creates a color point sequence which intersects the Planckian locus at a color temperature of 5000 K, typical of white light.
|
For further information: Prof. Dr. Willem Vos, University of Twente, Enschede, The Netherlands, email: [email protected] phone: +31-53-489-5388 or 5390.
|