Exploring the benefits and applications of self-assembly
As technology continues to advance, scientists and engineers are constantly searching for more efficient and effective ways to create new materials and devices. One increasingly popular method is self-assembly. This process involves the spontaneous organization of individual components into a larger, functional structure without the need for external control or intervention. In this article, we'll explore the various benefits, applications, challenges, and limitations associated with self-assembly, as well as how it can impact a wide range of industries.

Understanding Self-Assembly
Before diving in, it's important to have a clear understanding of what we mean by self-assembly. Simply put, this term refers to the process by which smaller components come together to form a larger, functional unit. This can include everything from the formation of protein complexes in living cells to the creation of nanoscale electronic devices in a laboratory.
Self-assembly is a fascinating process that occurs naturally in many different systems. For example, in living cells, proteins are able to self-assemble into complex structures that perform specific functions. This allows cells to carry out a wide range of processes, from generating energy to transmitting signals between cells.
The Core Concepts of Self-Assembly
At its core, self-assembly is all about the interactions between individual components. These interactions can be either physical (such as electrostatic forces) or chemical (such as the bonding between atoms or molecules). The goal is to take advantage of these natural interactions to bring the components together in a way that leads to the desired structure or function.
One important aspect of self-assembly is the role of entropy, which is a measure of the degree of disorder or randomness in a system. In self-assembly, striking a balance between the strength of the interactions between components and the system's entropy is crucial. If the components are too strongly attracted to one another, they may become "stuck" in one position and prevent the formation of the desired structure. Conversely, if the components are too disordered, they may not come together at all.
Key Principles and Types of Self-Assembly Processes
Successful self-assembly typically involves a careful balance between the strength of the interactions between components and the "entropy" of the system, as mentioned earlier. In other words, the components need to be able to come together in a way that is energetically favorable but not so strongly attracted to one another that they become "stuck" in one position.
There are two main types of self-assembly: "bottom-up" and "top-down." Bottom-up assembly involves starting with individual components and building up to a larger structure, while top-down assembly involves starting with a larger structure and breaking it down into smaller components. Both approaches have their pros and cons, depending on the desired outcome.
Bottom-up assembly is often used in nanotechnology, where scientists are working with extremely small components. By carefully controlling the interactions between individual atoms or molecules, they can build up complex structures with incredible precision.
Top-down assembly, on the other hand, is often used in fields like microelectronics, where larger structures need to be broken down into smaller components in order to achieve the desired functionality.
Applications of Self-Assembly in Various Industries
Self-assembly is a fascinating area of research that has the potential to transform a wide range of fields and industries. The ability of individual components to spontaneously come together and form complex structures has captured the imagination of scientists and engineers alike. In this section, we will examine the impact of self-assembly in nanotechnology and materials science, biomedical engineering, environmental solutions, and robotics and artificial intelligence.
Nanotechnology and Materials Science
One of the most promising applications of self-assembly is in the field of nanotechnology. Nanoscale structures created through self-assembly can be highly precise and uniform, with a wide range of potential uses. For example, self-assembled structures can be used as sensors, drug delivery vehicles, or even as components in next-generation electronic devices. Self-assembly can also be used to create new materials with unique properties, such as self-healing materials that can repair themselves when damaged.
Researchers are also exploring the use of self-assembly to create new types of nanomaterials. By combining different components in specific ways, they hope to create materials with novel properties and functions. For example, self-assembled nanomaterials could be used to create ultra-strong materials for use in aerospace or construction.
For instance, researchers realized that graphene – which possesses a variety of interesting properties like ultralow density, high compressibility, specific surface area, and electrical conductivity – could be an ideal building block to generate versatile 3D networks from controlled self-assembly. In their work, the team used graphene as the model building block, just like a Lego?? piece. By using a combination of 3D printed template and self-assembly technique, the graphene 'Lego pieces' can be assembled into a programmed structure from nano- to macro-scale.
Biomedical Engineering
Self-assembly also shows great promise in the field of biomedical engineering. By using self-assembly to create complex biomolecules such as DNA or proteins, researchers hope to develop new drugs, medical devices, and even artificial organs. Self-assembled structures can also be used to deliver drugs or gene therapy directly to targeted cells or tissues, potentially reducing the risk of side effects.
One area of particular interest is the use of self-assembly to create scaffolds for tissue engineering. By creating structures that mimic the natural environment of cells, researchers hope to create tissues and organs that can be transplanted into patients without the risk of rejection.
Scientists even managed to visualize self-assembly of peptides at unprecedented molecular resolution. They used frequency modulated atomic force microscopy to reveal the molecular architecture of a genetically designed peptide and its self-organization that forms single-molecule thick crystals on atomically flat graphite surfaces.
Environmental Solutions
Self-assembly also has potential applications in environmental science, particularly in the area of pollution remediation. By designing self-assembling materials that can capture and remove specific pollutants from the environment, researchers hope to develop more effective and efficient methods for cleaning up contaminated sites.
Another area of interest is the use of self-assembled materials for water purification. By creating materials that can selectively remove contaminants from water, researchers hope to develop new methods for providing clean drinking water to communities around the world.
Robotics and Artificial Intelligence
Finally, self-assembly has the potential to revolutionize the field of robotics and artificial intelligence. By using self-assembly to create complex, reconfigurable robot structures, researchers hope to develop machines that can adapt to changing environments, perform complex tasks, and even self-repair in the event of damage or malfunction.
Self-assembled robots could be used in a wide range of applications, from space exploration to disaster response. By creating robots that can assemble themselves on-site, researchers hope to reduce the cost and complexity of space missions and improve the speed and efficiency of disaster response efforts.
For instance, researchers have demonstrated flocks of self-replicating assembler robots show potential for making larger structures – although a fully autonomous self-replicating robot assembly system capable of both assembling larger structures, including larger robots, and planning the best construction sequence is still years away. But this work makes important strides toward that goal, including working out the complex tasks of when to build more robots and how big to make them, as well as how to organize swarms of bots of different sizes to build a structure efficiently without crashing into each other.

A conventional representation of a robot typically involves motors, circuits, and a metallic exterior. However, the emergence of molecular robotics is altering this perception. Similar to the formation of intricate living beings, molecular robots obtain their structure and functionality from assembled molecules. These robots have the potential to serve significant purposes, including diagnosing and treating diseases in vivo.
Constructing a molecular robot entails overcoming a fundamental challenge akin to the most elementary requirement of any organism: the body, which provides structural integrity. Nevertheless, fabricating elaborate structures, particularly on a microscopic scale, has been an engineering conundrum, with numerous constraints on what can currently be achieved.
In response to this issue, a group of researchers has devised a straightforward technique for constructing molecular robots using artificial, multicellular-like bodies. This approach employs molecules that can self-organize into the intended configuration.
A schematic diagram of how droplets extracted from a sponge self-assemble into a multicellular body structure. (Image: Richard Archer / Shin-Ichiro Nomura)
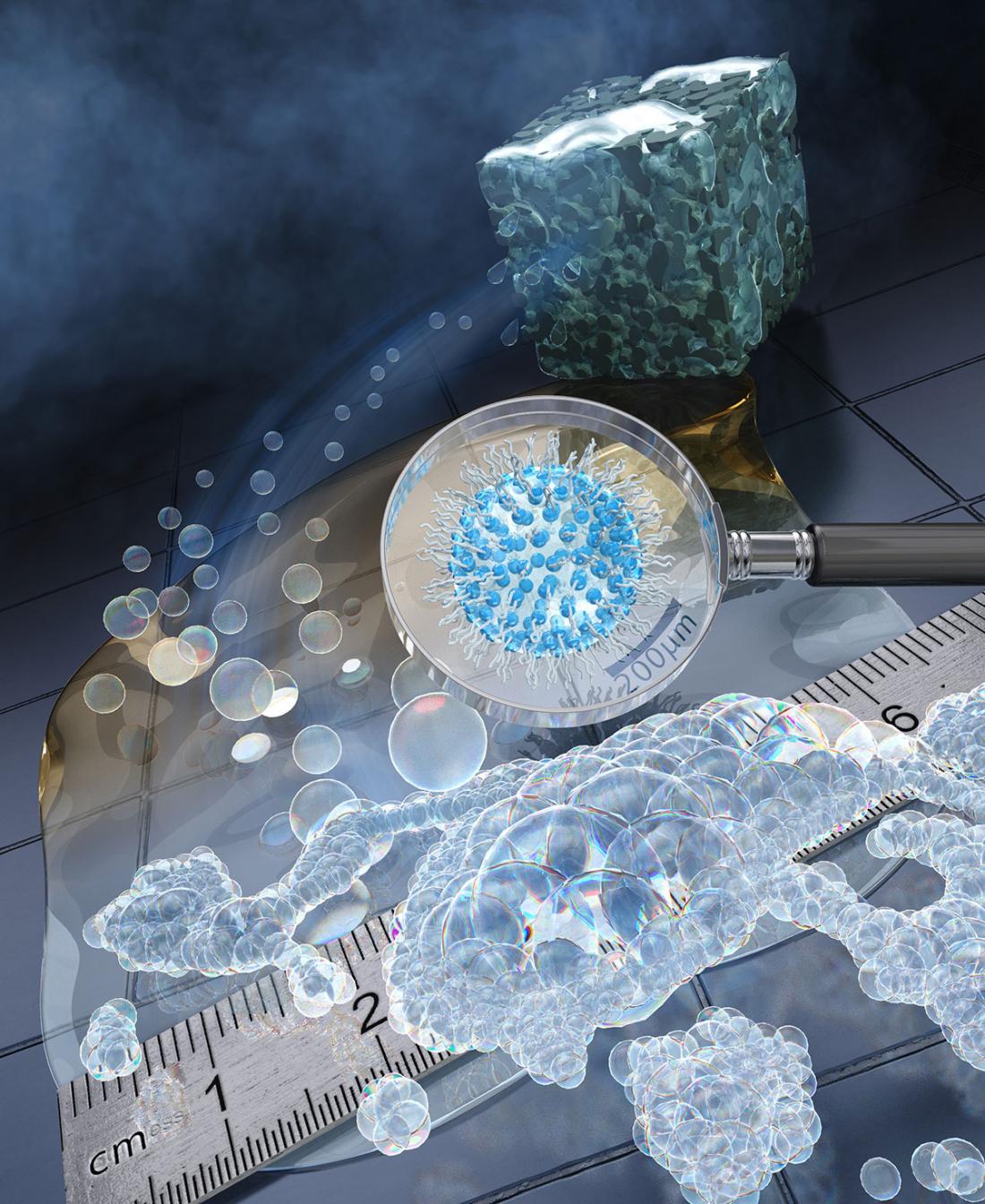
Advantages of Self-Assembly
Self-assembly is a fascinating field of research that has been gaining increasing attention in recent years. It involves the spontaneous organization of molecules or particles into complex structures, without the need for external intervention. Self-assembly has a wide range of potential applications, from drug delivery and tissue engineering to electronics and energy storage. Here are several key advantages that make it an attractive option for researchers and manufacturers alike:
Cost-Effectiveness
One major advantage of self-assembly is that it can be a highly cost-effective method of fabrication. Because it relies on natural interactions between components rather than expensive machinery or specialized equipment, it can often be less expensive and more accessible than other methods. This is especially true for the production of small-scale structures, where traditional manufacturing methods can be prohibitively expensive.
Furthermore, self-assembly can be performed in solution, which means that large quantities of material can be produced at once. This makes it an ideal method for industrial-scale production, where efficiency and cost-effectiveness are key considerations.
Scalability and Flexibility
Another advantage of self-assembly is its scalability and flexibility. Because it can be used to create structures on a wide range of scales - from the nanoscale to the macroscale - it has the potential to be applied to many different types of materials and devices. This makes it a highly versatile method of fabrication that can be tailored to suit a wide range of applications.
In addition, self-assembly can be performed under a variety of different conditions, including in solution, on surfaces, and in the gas phase. This means that it can be used to create structures in a wide range of environments, including in the human body. This flexibility makes self-assembly a highly adaptable method of fabrication that can be used to create a wide range of complex structures.
Enhanced Precision and Control
Perhaps one of the greatest advantages of self-assembly is the level of precision and control it offers. By carefully designing the interactions between components, researchers can create highly ordered and uniform structures with a high degree of accuracy. This can be especially useful for applications such as electronics or biomedical engineering, where precise control over the properties and behavior of materials is crucial.
Furthermore, self-assembly can be used to create structures with highly specific properties, such as porosity, conductivity, or magnetism. This level of control over the properties of materials is difficult to achieve using traditional manufacturing methods, making self-assembly a highly attractive option for researchers in a wide range of fields.
Environmental Sustainability
Finally, self-assembly has the potential to be a more environmentally sustainable method of fabrication. Because it does not rely on harsh chemicals or other toxic agents, it can be a greener alternative to traditional manufacturing methods that may produce harmful waste products or emissions.
In addition, self-assembly can be used to create materials with unique properties that may have environmental benefits. For example, self-assembled materials can be used to create highly efficient solar cells or to capture and store carbon dioxide from the atmosphere.
In conclusion, self-assembly is a highly promising method of fabrication that offers a wide range of advantages over traditional manufacturing methods. Its cost-effectiveness, scalability, flexibility, precision, and environmental sustainability make it a highly attractive option for researchers and manufacturers alike.
Challenges and Limitations of Self-Assembly
Of course, no technology is perfect, and self-assembly is no exception. There are several challenges and limitations associated with this method of fabrication that researchers and manufacturers need to be aware of:
Technical Difficulties and Complexities
Perhaps the biggest challenge with self-assembly is simply the technical difficulties and complexities involved. Because it relies on natural interactions between components, it can sometimes be difficult to control or predict the final outcome. Additionally, the process can be highly sensitive to factors such as temperature, pH, or the presence of other molecules, making it a potentially difficult process to reproduce on a large scale.
Reliability and Quality Control
Another challenge with self-assembly is ensuring reliable and consistent results. Because the process can be highly sensitive to small changes or variations, it can be difficult to ensure that every assembly is identical and performs as expected. This can be especially problematic for applications such as electronics, where even small defects or variations can have a significant impact on device performance.
Safety and Ethical Considerations
Finally, there are also safety and ethical considerations associated with self-assembly. Because the components used in self-assembly can be highly complex and novel, it can be difficult to predict their long-term effects on human health or the environment. Additionally, there may be ethical concerns associated with the creation of new materials or devices that have the potential to disrupt existing social or economic structures.
Conclusion
Despite these challenges, self-assembly remains an exciting and rapidly developing field of research. By taking advantage of the natural interactions between components, researchers and manufacturers hope to develop new materials and devices with a wide range of applications and benefits. Whether in the fields of nanotechnology, biomedical engineering, environmental solutions, or robotics and artificial intelligence, self-assembly has the potential to revolutionize the way we approach the design and fabrication of complex structures and materials.
As we continue to explore the possibilities of self-assembly, we can expect to see even more exciting developments in the years to come. By overcoming the challenges and limitations associated with this technology, scientists and engineers will be able to unlock the full potential of self-assembly, transforming a wide range of industries and improving our daily lives in the process.
From creating more effective and targeted drug delivery systems to designing adaptable robots capable of performing complex tasks in unpredictable environments, the possibilities are seemingly endless. While there is still much work to be done, self-assembly is poised to become an essential tool in our ongoing quest for technological advancement and innovation.
By understanding the benefits, applications, challenges, and limitations of self-assembly, as well as exploring the impact it can have on a wide range of industries, we can begin to harness its incredible potential. With continued research and development, self-assembly may soon play a critical role in shaping the future of technology and, ultimately, our world.