Flexoelectricity: Coupling Between Strain Gradients and Polarization in Solids
What is Flexoelectricity?
Flexoelectricity is a phenomenon in which a strain gradient in a material induces an electric polarization. It is a coupling between a mechanical strain gradient and an electric polarization in crystalline dielectrics, even in centrosymmetric materials that do not exhibit piezoelectricity. Flexoelectricity is a universal effect in all insulating materials, although its magnitude varies widely.
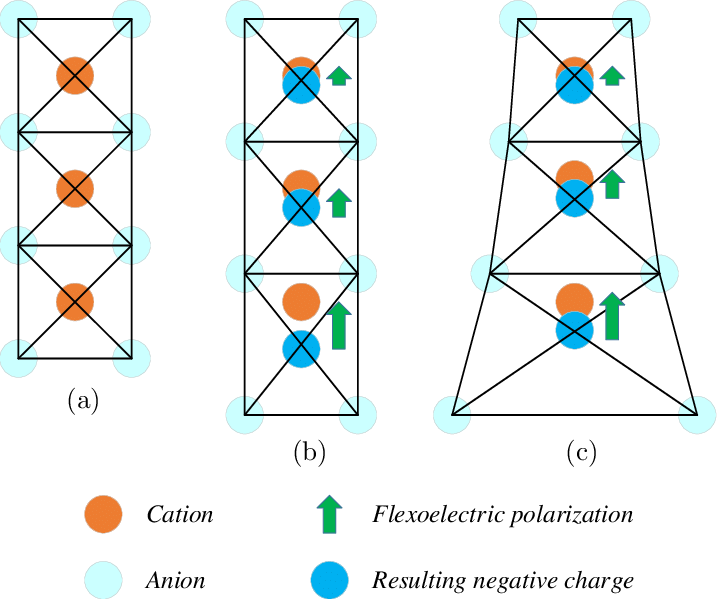
Origins of Flexoelectricity
Flexoelectricity arises from two main contributions:
- Bulk Flexoelectricity: This contribution originates from the distortion of the electron cloud around the atoms in the presence of a strain gradient. The induced polarization is proportional to the strain gradient and the flexoelectric coefficient tensor.
- Surface Flexoelectricity: This contribution comes from the change in the surface polarization due to the presence of a strain gradient. It is particularly relevant in nanoscale materials, where the surface-to-volume ratio is high.
Flexoelectric Effect in Nanomaterials
The flexoelectric effect is more pronounced in nanomaterials due to their high surface-to-volume ratio and the presence of large strain gradients. Some key aspects of flexoelectricity in nanomaterials include:
Size-Dependent Flexoelectricity
The flexoelectric effect becomes more significant as the size of the material decreases. In nanoscale materials, the strain gradients can be several orders of magnitude larger than in bulk materials, leading to enhanced flexoelectric responses. This size-dependent behavior opens up new possibilities for designing nanoscale electromechanical devices.
Flexoelectric Polarization in Nanostructures
Flexoelectricity can induce a significant polarization in various nanostructures, such as nanowires, nanotubes, and thin films. The flexoelectric polarization can be controlled by applying mechanical stress or by engineering the geometry and composition of the nanostructures. This control over polarization enables the development of novel nanoscale sensors, actuators, and energy harvesting devices.
Flexoelectric Coupling in Ferroelectrics
Flexoelectricity plays a crucial role in the behavior of ferroelectric materials at the nanoscale. The coupling between strain gradients and polarization can lead to the formation of exotic domain structures, such as polar vortices and skyrmions. These unique polarization states offer new functionalities for information storage and processing applications.
Applications of Flexoelectricity
Flexoelectricity has a wide range of potential applications in various fields:
Nanosensors and Actuators
The flexoelectric effect can be exploited to develop highly sensitive nanoscale sensors and actuators. Flexoelectric nanomaterials can convert mechanical deformations into electrical signals, enabling the detection of small forces, vibrations, and accelerations. Conversely, applying an electric field to a flexoelectric material can induce mechanical deformation, making it suitable for nanoscale actuation and positioning applications.
Energy Harvesting and Nanogenerators
Flexoelectric materials can be used to harvest mechanical energy from the environment and convert it into electrical energy. The strain gradients induced by vibrations, bending, or twisting can generate a flexoelectric polarization, which can be collected and stored in an electrical circuit. Flexoelectric energy harvesters, also known as nanogenerators, have the potential to power small electronic devices and sensors in remote or inaccessible locations. Nanogenerators based on flexoelectric nanomaterials, such as nanowires and nanotubes, have demonstrated high energy conversion efficiencies and the ability to harvest energy from a wide range of mechanical sources, including human motion, acoustic waves, and fluid flows.
Non-Volatile Memory
The coupling between strain gradients and polarization in flexoelectric materials can be utilized for non-volatile memory applications. By controlling the flexoelectric polarization states in nanoscale ferroelectric structures, information can be stored and retrieved in a non-volatile manner. Flexoelectric memory devices offer the advantages of low power consumption, high storage density, and fast switching speeds.
Challenges and Future Perspectives
Despite the significant progress in understanding and exploiting flexoelectricity, several challenges remain to be addressed. One of the main challenges is the accurate measurement and characterization of flexoelectric coefficients in nanomaterials. The development of standardized measurement techniques and the establishment of reliable databases for flexoelectric properties are crucial for the advancement of the field.
Future research directions in flexoelectricity include the exploration of new flexoelectric materials with enhanced properties, the optimization of device architectures for specific applications, and the integration of flexoelectric nanomaterials with other functional materials and devices. The combination of flexoelectricity with other coupled phenomena, such as piezoelectricity and ferroelectricity, can lead to the development of multifunctional and adaptive nanoscale systems.
Further Reading
Progress in Materials Science, Flexoelectricity in Solids: Progress, Challenges, and Perspectives
Nanotechnology, Fundamentals of flexoelectricity in solids