Beyond Rayleigh: Mie Scattering and Its Importance for Larger Nanoparticles
What is Mie Scattering?
Mie scattering is a phenomenon that describes the elastic scattering of electromagnetic radiation by particles that have sizes comparable to or larger than the wavelength of the incident light. It is named after Gustav Mie, a German physicist who first developed a comprehensive theory of this scattering process in 1908. Mie scattering is a fundamental concept in nanoscience and nanotechnology, as it governs the optical properties of nanoparticles and their interactions with light.
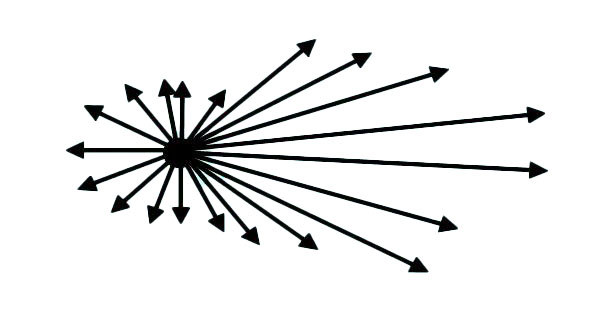
Key Concepts in Mie Scattering
Mie scattering involves several key concepts that are essential for understanding the interaction between light and particles:
Particle Size and Wavelength
The scattering behavior of a particle depends on its size relative to the wavelength of the incident light. When the particle size is much smaller than the wavelength (typically less than 1/10 of the wavelength), the scattering is described by Rayleigh scattering, which is a simpler case of Mie scattering. As the particle size becomes comparable to or larger than the wavelength, Mie scattering becomes dominant, and the scattering behavior becomes more complex.
Refractive Index
The refractive index of a particle, which is a measure of how much the particle slows down light relative to its speed in vacuum, plays a crucial role in Mie scattering. The difference in refractive index between the particle and the surrounding medium determines the strength and angular distribution of the scattered light. Particles with a higher refractive index contrast scatter light more strongly than those with a lower contrast.
Scattering Intensity and Angular Distribution
Mie scattering results in a complex angular distribution of the scattered light, with the intensity varying as a function of the scattering angle. The scattering intensity is typically highest in the forward direction (i.e., in the same direction as the incident light) and decreases with increasing scattering angle. The exact angular distribution depends on the size, shape, and optical properties of the particle, as well as the wavelength of the incident light.
Mie Theory
Mie theory is a mathematical framework that provides a rigorous solution to the problem of light scattering by spherical particles. It is based on solving Maxwell's equations for the electromagnetic fields inside and outside the particle, taking into account the boundary conditions at the particle surface. Mie theory enables the calculation of the scattering and absorption cross-sections of a particle, as well as the angular distribution of the scattered light.
The key assumptions of Mie theory are:
- The particle is spherical and homogeneous
- The particle and the surrounding medium are isotropic and non-magnetic
- The incident light is a plane wave
While Mie theory provides an exact solution for spherical particles, it can also be extended to approximate the scattering by non-spherical particles using techniques such as the T-matrix method or the discrete dipole approximation.
Applications of Mie Scattering
Mie scattering has numerous applications in nanoscience and nanotechnology, as well as in fields such as atmospheric science, colloidal chemistry, and biophysics. Some key applications include:
Nanomaterials Characterization
Mie scattering is widely used to characterize the size, shape, and optical properties of nanoparticles. Techniques such as dynamic light scattering (DLS) and UV-visible spectroscopy rely on Mie scattering to determine the size distribution and absorption/scattering spectra of nanoparticle suspensions.
Plasmonics and Nanophotonics
Mie scattering plays a central role in the field of plasmonics, which studies the collective oscillations of free electrons in metallic nanostructures. Plasmonic nanoparticles exhibit strong Mie scattering at specific wavelengths, enabling applications such as surface-enhanced Raman spectroscopy (SERS), photonic circuits, and optical metamaterials.
Atmospheric Science and Remote Sensing
Mie scattering is essential for understanding the optical properties of atmospheric aerosols, such as dust, smoke, and pollution particles. It is used in remote sensing techniques, such as lidar and satellite imaging, to monitor the concentration and distribution of aerosols in the atmosphere and their impact on climate and air quality.
Biomedical Applications
Mie scattering is exploited in various biomedical applications, such as optical imaging, biosensing, and drug delivery. Nanoparticles designed to scatter light at specific wavelengths can be used as contrast agents for imaging techniques like optical coherence tomography (OCT) and confocal microscopy. Mie scattering also plays a role in the optical properties of biological tissues and cells.
Challenges and Future Perspectives
Despite the well-established theory and numerous applications of Mie scattering, several challenges remain in its practical implementation. One of the main challenges is the accurate characterization of non-spherical and inhomogeneous particles, which require more advanced computational methods and experimental techniques.
Future research in Mie scattering will focus on the development of novel nanostructures with tailored scattering properties, such as high directionality, wavelength selectivity, and polarization control. The integration of Mie scattering with other nanoscale phenomena, such as surface plasmon resonance and quantum confinement, will lead to new opportunities in areas like nanoscale sensing, imaging, and energy harvesting.
Additionally, the application of machine learning and data-driven approaches to Mie scattering will enable the rapid design and optimization of nanostructures for specific applications, accelerating the discovery and development of novel nanomaterials and devices.
Further Reading
Bulletin of the American Meteorological Society, Gustav Mie and the evolving discipline of electromagnetic scattering by particles
Dielectric Metamaterials, Fundamentals of Mie scattering
Laser & Photonics Reviews, Mie Scattering for Photonic Devices