Pseudocapacitors: Bridging the Gap Between Batteries and Capacitors
What are Pseudocapacitors?
Pseudocapacitors are a type of electrochemical energy storage device that combines the high energy density of batteries with the fast charge/discharge rates and long cycle life of capacitors. They store energy through fast and reversible surface or near-surface redox reactions, resulting in capacitive behavior. Pseudocapacitors are also known as redox supercapacitors or faradaic supercapacitors.
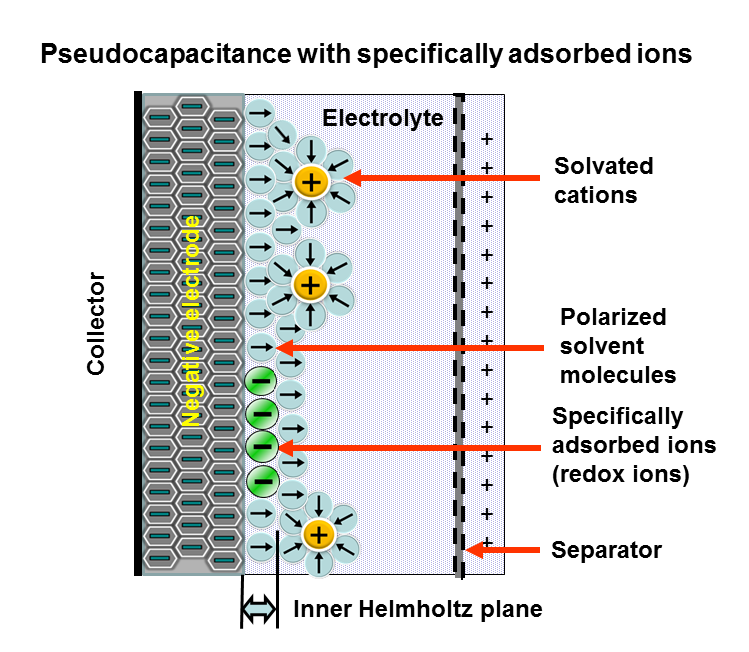
Key Characteristics of Pseudocapacitors
Pseudocapacitors exhibit several unique characteristics that distinguish them from conventional capacitors and batteries:
- High Energy Density: Pseudocapacitors can store significantly more energy than electric double-layer capacitors (EDLCs) due to the additional charge storage from redox reactions. Their energy density is typically higher than that of conventional capacitors and approaching that of batteries.
- Fast Charge/Discharge Rates: The fast surface or near-surface redox reactions in pseudocapacitors enable rapid charge and discharge processes, similar to those of capacitors. This allows for high power density and quick response times.
- Long Cycle Life: Pseudocapacitors can undergo a large number of charge/discharge cycles without significant degradation, thanks to the reversible nature of the redox reactions. They often exhibit longer cycle life compared to batteries.
Pseudocapacitive Materials
The performance of pseudocapacitors heavily relies on the choice of electrode materials. Ideal pseudocapacitive materials should have high surface area, good electrical conductivity, and reversible redox behavior. Some common pseudocapacitive materials include:
Transition Metal Oxides
Transition metal oxides, such as ruthenium oxide (RuO2), manganese oxide (MnO2), and vanadium oxide (V2O5), are widely studied as pseudocapacitive materials. These oxides exhibit multiple oxidation states and can undergo fast and reversible redox reactions, leading to high specific capacitance.
Conducting Polymers
Conducting polymers, such as polyaniline (PANI), polypyrrole (PPy), and poly(3,4-ethylenedioxythiophene) (PEDOT), are another class of pseudocapacitive materials. They store charge through reversible doping/dedoping processes accompanied by ion intercalation. Conducting polymers offer high conductivity, flexibility, and low cost.
Metal Hydroxides and Sulfides
Metal hydroxides, such as nickel hydroxide (Ni(OH)2) and cobalt hydroxide (Co(OH)2), and metal sulfides, like molybdenum disulfide (MoS2), have also shown promising pseudocapacitive behavior. These materials undergo reversible redox reactions and offer high theoretical capacitance.
Nanostructuring in Pseudocapacitors
Nanostructuring plays a crucial role in enhancing the performance of pseudocapacitive materials. By designing materials with nanoscale features, such as nanoparticles, nanowires, and nanosheets, several benefits can be achieved:
- High Surface Area: Nanostructured materials possess a large surface area, which increases the number of active sites for redox reactions, leading to higher specific capacitance.
- Short Ion Diffusion Paths: The nanoscale dimensions of the materials shorten the ion diffusion paths, enabling faster charge/discharge rates and improved power density.
- Enhanced Electrical Conductivity: Nanostructuring can improve the electrical conductivity of pseudocapacitive materials by creating interconnected networks and reducing the resistance for electron transport.
Applications of Pseudocapacitors
Pseudocapacitors find applications in various fields where high power density, fast charge/discharge, and long cycle life are required:
Portable Electronics
Pseudocapacitors can be used in portable electronic devices, such as smartphones, tablets, and wearables, to provide quick charging and longer battery life. They can also be used in combination with batteries to handle peak power demands.
Electric Vehicles
Pseudocapacitors can complement batteries in electric vehicles, providing high power for acceleration and regenerative braking. They can also help extend the battery life by reducing the stress on the battery during high power events.
Grid Energy Storage
Pseudocapacitors can be used in grid-scale energy storage systems to provide fast frequency regulation and smooth out power fluctuations from renewable energy sources like wind and solar.
Challenges and Future Perspectives
Despite the promising potential of pseudocapacitors, several challenges need to be addressed for their widespread adoption. One of the main challenges is the limited energy density compared to batteries. Researchers are exploring new pseudocapacitive materials and hybrid systems to further increase the energy density while maintaining high power density and long cycle life.
Another challenge is the cost and scalability of pseudocapacitive materials. Many high-performance materials, such as ruthenium oxide, are expensive and scarce. Efforts are being made to develop low-cost alternatives and improve the manufacturing processes for large-scale production.
Future research directions in pseudocapacitors include the development of novel pseudocapacitive materials, the optimization of electrode architectures, and the integration of pseudocapacitors with other energy storage and conversion devices. The combination of computational modeling, in situ characterization techniques, and advanced manufacturing methods will play a crucial role in advancing the field of pseudocapacitors.
Further Reading
National Science Review, Pseudocapacitive materials for electrochemical capacitors: from rational synthesis to capacitance optimization
Accounts of Materials Research, Two-Dimensional Pseudocapacitive Nanomaterials for High-Energy- and High-Power-Oriented Applications of Supercapacitors