Atom Probe Tomography: Uncovering the 3D Atomic Structure of Materials
What is Atom Probe Tomography?
Atom Probe Tomography (APT) is a powerful analytical technique that provides three-dimensional (3D) imaging and chemical composition analysis of materials at the atomic scale. It combines the principles of field ion microscopy and mass spectrometry to map the positions and identities of individual atoms within a sample, enabling researchers to unravel the complex nanostructures and chemical distributions in a wide range of materials, from metals and semiconductors to biological specimens.
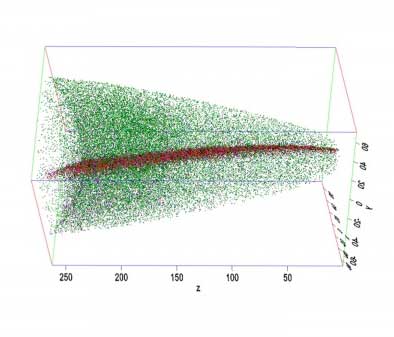
How Does Atom Probe Tomography Work?
The APT technique involves the following key steps:
- Sample Preparation: The material to be analyzed is fabricated into a sharp needle-shaped specimen with a tip radius of approximately 50 nm. This is typically achieved using focused ion beam milling or electropolishing techniques.
- Field Evaporation: The specimen is placed in an ultra-high vacuum chamber and cooled to cryogenic temperatures (20-100 K). A high positive voltage (2-15 kV) is applied to the specimen, creating an intense electric field at the tip. This field causes the controlled evaporation and ionization of atoms from the surface of the specimen.
- Ion Detection: The evaporated ions are accelerated away from the specimen by the electric field and projected onto a position-sensitive detector, typically a microchannel plate (MCP) or delay-line detector (DLD). The time-of-flight of each ion is measured, allowing for the determination of its mass-to-charge ratio and, consequently, its chemical identity.
- Data Reconstruction: The collected data, including the positions and chemical identities of the detected ions, are used to reconstruct a 3D atom-by-atom map of the specimen. Sophisticated data processing algorithms, such as the protocol for spatial and chemical reconstruction (POSAP) or the corrective-evolutional reconstruction (CER) method, are employed to convert the raw data into a 3D point cloud representing the atomic structure and composition of the material.
Advantages of Atom Probe Tomography
APT offers several unique advantages over other nanoscale characterization techniques:
- Atomic Resolution: APT provides true atomic-scale resolution in three dimensions, with a spatial resolution of up to 0.1-0.3 nm in depth and 0.3-0.5 nm laterally. This allows for the direct visualization and analysis of individual atoms and their local environments.
- Chemical Sensitivity: APT can detect all elements in the periodic table, including light elements such as hydrogen and lithium, which are challenging to analyze with other techniques. It provides quantitative information on the chemical composition of the sample with a sensitivity of up to 10 parts per million (ppm).
- 3D Imaging: Unlike two-dimensional (2D) characterization techniques, such as transmission electron microscopy (TEM) or scanning probe microscopy (SPM), APT enables the reconstruction of the complete 3D atomic structure of the material. This is particularly valuable for understanding the complex nanostructures, interfaces, and defects in materials.
- Correlative Analysis: APT data can be combined with complementary characterization techniques, such as TEM, scanning electron microscopy (SEM), or X-ray diffraction (XRD), to gain a more comprehensive understanding of the material's structure and properties. This correlative approach allows for the validation and interpretation of APT results in the context of other nanoscale information.
Applications of Atom Probe Tomography
APT has found applications in a wide range of fields, including:
Materials Science and Engineering
APT is extensively used in the characterization of advanced materials, such as nanostructured alloys, semiconductors, and ceramics. It provides valuable insights into the atomic-scale distribution of elements, precipitation phenomena, segregation at interfaces and grain boundaries, and the formation of nanoscale phases. This information is crucial for understanding the structure-property relationships in materials and guiding the development of new materials with tailored properties.
Semiconductor Industry
APT plays a significant role in the semiconductor industry, particularly in the analysis of advanced nanoelectronic devices and the optimization of fabrication processes. It enables the characterization of dopant distributions, interface chemistry, and the 3D structure of semiconductor heterostructures and nanodevices. APT has been instrumental in the development of novel materials and device architectures for high-performance electronics.
Energy Storage and Conversion
APT is increasingly applied in the field of energy storage and conversion, particularly in the characterization of battery materials, fuel cells, and catalysts. It provides insights into the nanoscale distribution of elements, the formation of solid-electrolyte interphases (SEI), and the degradation mechanisms in lithium-ion batteries. APT also helps in understanding the atomic-scale structure and composition of catalytic nanoparticles and their role in energy conversion processes.
Biological and Life Sciences
Although APT has primarily been used for the characterization of inorganic materials, recent advancements have extended its application to biological specimens. APT has been used to study the 3D atomic structure of proteins, the distribution of elements in cells and tissues, and the composition of biominerals. This emerging field, known as "Atom Probe Tomography for Biology," opens up new possibilities for understanding the structure and function of biological systems at the atomic scale.
Challenges and Future Perspectives
Despite the remarkable capabilities of APT, there are still challenges and limitations to be addressed. Sample preparation remains a critical aspect, as the fabrication of suitable needle-shaped specimens can be time-consuming and requires expertise. Moreover, the field evaporation process can introduce artifacts, such as preferential evaporation or surface migration of atoms, which need to be carefully considered during data interpretation.
Future developments in APT are aimed at improving the spatial and chemical resolution, increasing the data acquisition rate, and enhancing the ease of use. The integration of APT with other in-situ characterization techniques, such as TEM or environmental SEM, will enable real-time observation of dynamic processes at the atomic scale. Furthermore, advancements in data processing and visualization tools will facilitate the efficient handling and interpretation of the large datasets generated by APT.
As APT continues to evolve and expand its applications, it will play an increasingly important role in the advancement of nanoscience and nanotechnology. By providing unprecedented insights into the 3D atomic structure and chemical composition of materials, APT will contribute to the development of new materials, devices, and technologies that address critical challenges in energy, electronics, and healthcare.
Further Reading
Nature Reviews Methods Primers, Atom probe tomography
Materials Today Advances, New frontiers in atom probe tomography: a review of research enabled by cryo and/or vacuum transfer systems
Microstructures, Cryogenic atom probe tomography and its applications: a review