Quantum Spin Hall Insulator: A New State of Matter for Spintronics and Quantum Computing
What is a Quantum Spin Hall Insulator?
A quantum spin Hall insulator (QSHI) is a novel state of matter that exhibits unique electronic properties. It is a type of topological insulator, which is a material that behaves as an insulator in its interior but has conducting states on its surface. In a QSHI, these surface states are spin-polarized, meaning that electrons with opposite spins move in opposite directions along the edges of the material, forming a "edge channel". This phenomenon is known as the quantum spin Hall effect.
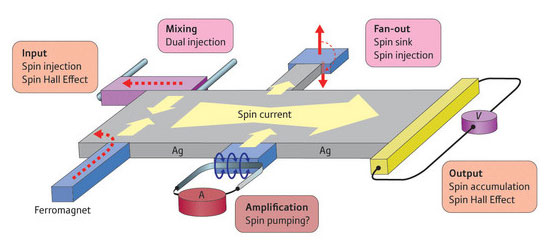
Key Properties of QSHIs
QSHIs possess several unique properties that make them promising for applications in spintronics and quantum computing:
- Spin-Polarized Edge Channels: The edge channels in a QSHI are spin-polarized, meaning that electrons with opposite spins move in opposite directions. This spin-polarization is a result of strong spin-orbit coupling in the material.
- Topological Protection: The spin-polarized edge channels in a QSHI are topologically protected, which means that they are robust against backscattering and impurities. This protection arises from the topology of the electronic band structure and ensures that the edge channels remain conducting even in the presence of defects or disorder.
- Dissipationless Transport: The topological protection of the edge channels also leads to dissipationless transport, meaning that electrons can flow without losing energy. This property is highly desirable for low-power electronic devices and could enable more efficient information processing.
Materials Exhibiting QSHI Behavior
Several materials have been predicted and experimentally verified to exhibit QSHI behavior. Some notable examples include:
HgTe/CdTe Quantum Wells
Mercury telluride (HgTe) and cadmium telluride (CdTe) quantum wells were the first systems in which the quantum spin Hall effect was observed experimentally. By tuning the thickness of the HgTe layer, researchers can control the band structure and induce a topological phase transition, leading to the formation of spin-polarized edge channels.
Bismuth-Based Compounds
Bismuth-based compounds, such as bismuth selenide (Bi2Se3) and bismuth telluride (Bi2Te3), have been extensively studied as QSHIs. These materials have strong spin-orbit coupling and a large bandgap, making them suitable for room-temperature applications.
Transition Metal Dichalcogenides
Transition metal dichalcogenides (TMDs), such as tungsten diselenide (WSe2) and molybdenum disulfide (MoS2), have also been predicted to exhibit QSHI behavior. These materials have a layered structure and can be exfoliated into thin sheets, enabling the exploration of quantum spin Hall effect in two-dimensional systems.
Applications of QSHIs
QSHIs have potential applications in various fields, including spintronics and quantum computing:
Spintronics
QSHIs can be used as building blocks for spintronic devices, which utilize the spin degree of freedom of electrons for information processing. The spin-polarized edge channels in QSHIs can serve as spin-polarized current sources or spin filters, enabling efficient spin injection and manipulation. This could lead to the development of low-power, high-speed spintronic devices for data storage and processing.
Quantum Computing
QSHIs also hold promise for quantum computing applications. The topologically protected edge channels can be used to create quantum bits (qubits) that are resistant to decoherence and errors. By exploiting the spin-polarized nature of these edge channels, researchers can develop robust quantum gates and circuits for fault-tolerant quantum computation.
Challenges and Future Perspectives
Despite the exciting prospects of QSHIs, several challenges need to be addressed for their practical implementation. One of the main challenges is the fabrication of high-quality QSHI materials with well-defined edge channels. The presence of impurities, defects, or edge roughness can disrupt the topological protection and degrade the performance of QSHI-based devices.
Future research in QSHIs will focus on the discovery and characterization of new QSHI materials with improved properties, such as larger bandgaps, higher spin-orbit coupling, and better compatibility with existing semiconductor technologies. The integration of QSHIs with other quantum materials, such as superconductors and magnetic materials, will also be explored to create hybrid quantum devices with enhanced functionality.
Further Reading
Journal of the Physical Society of Japan, The Quantum Spin Hall Effect: Theory and Experiment
Advanced Materials, Atomically Thin Quantum Spin Hall Insulators