Giant Magnetoresistance: A Quantum Leap in Data Storage
What is Giant Magnetoresistance?
Giant magnetoresistance (GMR) is a quantum mechanical effect observed in thin film structures composed of alternating ferromagnetic and non-magnetic layers. It is characterized by a significant change in electrical resistance in response to an applied magnetic field. The discovery of GMR in the late 1980s revolutionized the field of data storage and earned the Nobel Prize in Physics for Albert Fert and Peter Grünberg in 2007.
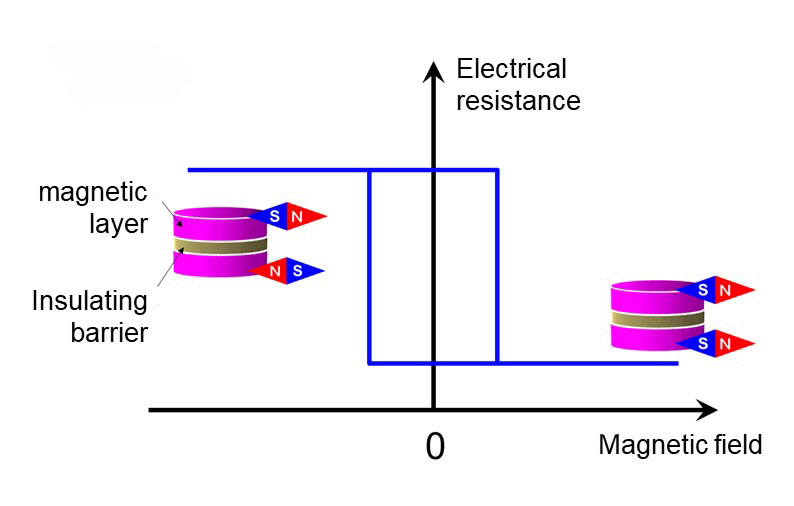
Mechanism of Giant Magnetoresistance
The GMR effect arises from the spin-dependent scattering of conduction electrons in the thin film structure. The key components of a GMR system are:
- Ferromagnetic Layers: These layers, typically made of materials like iron, cobalt, or their alloys, have a net magnetic moment due to the alignment of electron spins.
- Non-Magnetic Layers: Thin layers of non-magnetic materials, such as copper or chromium, separate the ferromagnetic layers and mediate the exchange coupling between them.
In the absence of an external magnetic field, the magnetic moments of the ferromagnetic layers are anti-parallel due to the interlayer exchange coupling. This anti-parallel alignment causes enhanced scattering of the conduction electrons, as electrons with spins aligned parallel to the magnetization of a layer can pass through easily, while those with opposite spins are scattered strongly. The result is a high electrical resistance state.
When an external magnetic field is applied, the magnetic moments of the ferromagnetic layers align parallel to each other. In this configuration, electrons with either spin orientation can pass through the layers more easily, leading to a significant decrease in electrical resistance. The relative change in resistance between the anti-parallel and parallel states can be as high as 50% or more, hence the term "giant" magnetoresistance.
Applications of Giant Magnetoresistance
The GMR effect has found extensive applications in various fields, most notably in data storage and sensing:
Hard Disk Drives
GMR-based read heads have revolutionized the hard disk drive industry. The high sensitivity of GMR sensors to magnetic fields allows for the detection of smaller magnetic bits on the disk surface, enabling higher data storage densities. GMR read heads have facilitated the tremendous increase in hard disk drive capacities over the past few decades.
Magnetic Field Sensors
GMR sensors are widely used for precise magnetic field measurements in various applications, such as:
- Automotive systems: wheel speed sensors, electronic compasses
- Industrial automation: position and motion sensors
- Biomedical devices: biosensors for magnetic nanoparticle detection
Spintronic Devices
GMR is a fundamental building block of spintronics, an emerging field that exploits the spin degree of freedom of electrons for information processing. GMR-based spintronic devices, such as spin valves and magnetic tunnel junctions, are being explored for applications in non-volatile memory (MRAM), logic circuits, and neuromorphic computing.
Advances in Giant Magnetoresistance
Since the discovery of GMR, researchers have made significant progress in understanding and optimizing the effect. Some notable advancements include:
- Material Optimization: Exploration of new ferromagnetic and non-magnetic materials, as well as engineering of interfaces, has led to enhanced GMR ratios and improved device performance.
- Tunneling Magnetoresistance (TMR): By replacing the non-magnetic metallic layer with a thin insulating barrier, even higher magnetoresistance ratios can be achieved through the quantum mechanical tunneling of electrons. This effect, known as tunneling magnetoresistance, is utilized in magnetic tunnel junctions for advanced spintronic applications.
- Spin Transfer Torque (STT): The discovery of spin transfer torque has enabled the electrical control of magnetization in GMR structures. STT allows for the writing of information in magnetic memory devices using spin-polarized currents, paving the way for high-density and low-power spintronic memory.
Future Perspectives
Giant magnetoresistance continues to be a fertile ground for research and innovation. Future directions in GMR research include:
- Development of novel GMR-based sensors with improved sensitivity, speed, and miniaturization.
- Integration of GMR structures with other emerging technologies, such as 2D materials and topological insulators, for enhanced functionality and performance.
- Exploration of GMR in unconventional geometries, such as nanowires and nanoparticles, for novel spintronic devices and applications.
As the demand for high-density data storage, energy-efficient computing, and smart sensors continues to grow, giant magnetoresistance will undoubtedly play a crucial role in shaping the future of these technologies.