Heterostructure Catalysis: Unlocking New Catalytic Possibilities
What is Heterostructure Catalysis?
Heterostructure catalysis is an emerging field that focuses on the design and application of catalytic materials composed of two or more distinct components with different chemical compositions, crystal structures, or electronic properties. By carefully engineering the interfaces between these components at the nanoscale, heterostructure catalysts can exhibit unique catalytic properties and enhanced performance compared to their individual components.
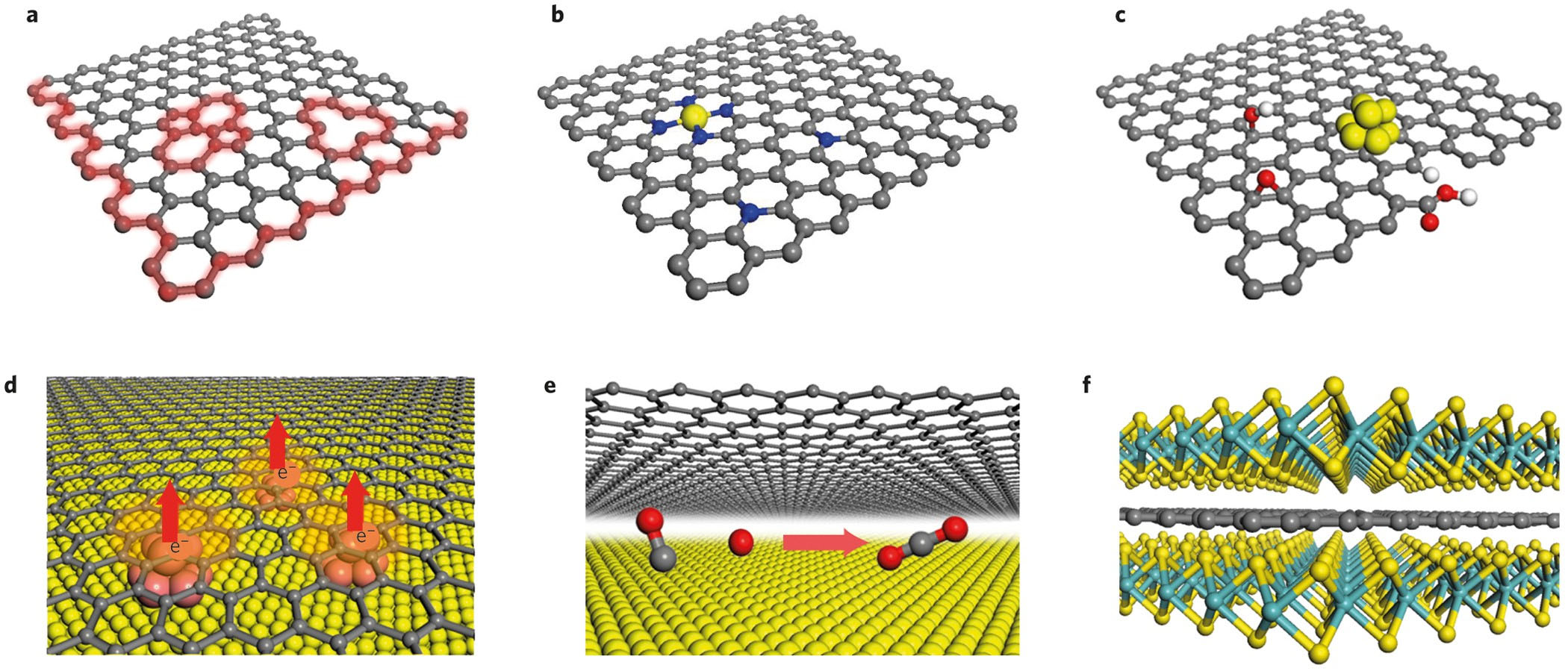
Key Concepts in Heterostructure Catalysis
Heterostructure catalysis relies on several key concepts that distinguish it from traditional catalysis:
- Interfacial Effects: The interfaces between the different components in a heterostructure catalyst play a crucial role in determining its catalytic properties. These interfaces can facilitate charge transfer, modify the electronic structure of the catalyst, and create unique active sites for catalytic reactions.
- Synergistic Effects: Heterostructure catalysts can exhibit synergistic effects, where the catalytic performance of the combined materials is greater than the sum of their individual parts. This synergy arises from the beneficial interactions between the different components, such as charge redistribution, strain effects, or the formation of new active sites.
- Tunable Properties: By carefully selecting the components and engineering their interfaces, researchers can tune the catalytic properties of heterostructure catalysts. This tunability allows for the optimization of catalytic activity, selectivity, and stability for specific reactions and applications.
Types of Heterostructure Catalysts
Heterostructure catalysts can be classified into several types based on the nature of their components and the way they are combined:
Metal-Metal Heterostructures
Metal-metal heterostructures consist of two or more different metal nanoparticles or nanosheets combined to form a catalytic material. These heterostructures can exhibit enhanced catalytic activity and selectivity due to the synergistic effects between the metal components, such as electronic coupling, strain effects, or the formation of alloy interfaces.
Metal-Support Heterostructures
Metal-support heterostructures are composed of metal nanoparticles deposited on a support material, such as a metal oxide, carbon-based material, or zeolite. The interface between the metal and the support can modify the electronic structure of the metal, facilitate charge transfer, and provide additional active sites for catalytic reactions.
Semiconductor-Semiconductor Heterostructures
Semiconductor-semiconductor heterostructures combine two or more semiconductor materials with different band gaps, crystal structures, or electronic properties. These heterostructures can enhance photocatalytic activity by improving charge separation, extending light absorption, or facilitating charge transfer between the components.
Synthesis and Characterization of Heterostructure Catalysts
The synthesis and characterization of heterostructure catalysts are crucial aspects of their development and optimization. Various synthesis methods have been employed to create heterostructure catalysts, including:
- Wet-Chemical Methods: Wet-chemical methods, such as co-precipitation, sol-gel, and hydrothermal synthesis, allow for the controlled growth and assembly of heterostructure components. These methods enable the tuning of size, shape, and composition of the individual components and their interfaces.
- Physical Deposition Methods: Physical deposition methods, such as sputtering, evaporation, and pulsed laser deposition, can be used to create heterostructure catalysts with precise control over the thickness and composition of the individual layers. These methods are particularly useful for creating thin film heterostructures.
- Surface Modification Techniques: Surface modification techniques, such as atomic layer deposition (ALD) and chemical vapor deposition (CVD), can be employed to create heterostructures by depositing one material onto the surface of another. These techniques allow for the precise control over the thickness and composition of the deposited layer.
The characterization of heterostructure catalysts is essential for understanding their structure-property relationships and optimizing their performance. Various techniques are used to probe the composition, morphology, electronic structure, and catalytic properties of heterostructure catalysts, including:
- Electron microscopy (SEM, TEM, STEM)
- X-ray diffraction (XRD)
- X-ray photoelectron spectroscopy (XPS)
- Raman spectroscopy
- Temperature-programmed reduction/oxidation (TPR/TPO)
- Catalytic activity and selectivity measurements
Applications of Heterostructure Catalysts
Heterostructure catalysts have shown promising performance in various catalytic applications, including:
Renewable Energy
Heterostructure catalysts can be used in renewable energy applications, such as water splitting for hydrogen production, CO2 reduction to fuels and chemicals, and fuel cells. The synergistic effects and enhanced charge transfer in heterostructure catalysts can improve the efficiency and selectivity of these reactions.
Environmental Remediation
Heterostructure catalysts can be applied in environmental remediation processes, such as the degradation of organic pollutants, the reduction of toxic gases (e.g., NOx, SOx), and the removal of heavy metals from water. The unique properties of heterostructure catalysts can enhance the catalytic activity and stability in these challenging environments.
Chemical Synthesis
Heterostructure catalysts can be employed in the selective synthesis of valuable chemicals, such as pharmaceuticals, fine chemicals, and polymers. The tunable properties of heterostructure catalysts can enable the selective conversion of substrates to desired products, reducing the formation of byproducts and improving the overall efficiency of the process.
Challenges and Future Perspectives
Despite the significant progress in heterostructure catalysis, several challenges need to be addressed for their widespread application. One of the main challenges is the scalable and cost-effective synthesis of heterostructure catalysts with precise control over their composition, morphology, and interfaces. The long-term stability and durability of heterostructure catalysts under realistic operating conditions also need to be improved.
Future research in heterostructure catalysis will focus on the rational design and synthesis of novel heterostructure catalysts with tailored properties for specific applications. The integration of computational modeling and machine learning techniques will accelerate the discovery and optimization of heterostructure catalysts. Additionally, the development of in situ and operando characterization techniques will provide deeper insights into the structure-property relationships and reaction mechanisms of heterostructure catalysts under working conditions.
Further Reading
Advanced Functional Materials, Heterostructured Catalysts for Electrocatalytic and Photocatalytic Carbon Dioxide Reduction