Dec 14, 2015 |
Biophysicists discover the key feature of an accurate tool for optogenetics
|
(Nanowerk News) Biophysicists have developed a hypothesis to explain the function of a light-driven protein which pumps sodium ions across a cell membrane, and they have revealed the key structural feature of these pumps. The scientists see these sodium pumps as being highly promising tools in using light signals to control nerve cells – which is exactly what is involved in the new scientific field of optogenetics.
|
The paper written by specialists from Mosow Institute of Physics and Technology (MIPT), with their German and French colleagues from Research Centre Jülich, Max Planck Institute of Biophysics and Institut de Biologie Structurale, has been published in the magazine FEBS Letters ("Structure of the light-driven sodium pump KR2 and its implications for optogenetics").
|
Research in the field of optogenetics has been of great interest to scientists over the past ten years. One of the many applications of the new science is working with individual cells of the nervous system, in order to study various types of neurodegenerative diseases (such as Alzheimer’s or Parkinson’s). Studies have also been conducted that demonstrate that optogenetic methods can be used to restore vision in mice. In this paper, the scientists studied the membrane protein KR2 – it is found in cell membranes and when exposed to light it is able to transport sodium ions. Let us look in a little more detail at how a charged particle passing across a cell membrane is connected with nerve impulses.
|
Meanwhile, at the cell membrane…
|
Among the multitude of proteins (approximately 20,000) encoded in the human genome, membrane proteins are grouped in a separate category. They are complex molecular mechanisms that are embedded in the membrane of every living cell and perform a variety of functions. Some of them act as active carriers of substances that are not able to pass through a cell membrane themselves – e.g. chlorine or potassium ions, which a cell uses to regulate its internal electrolyte balance.
|
In the human body, nerve cells use these ions to transmit nerve impulses: when charged particles are transferred through the membrane of a neuron, the potential difference changes on either side of it and the electrical signal is passed on. In order to allow the following signal to pass through, the neuron has to “reset” the potential to its initial value. To do this, it uses special molecular mechanisms that pump ions out – ion pumps
|
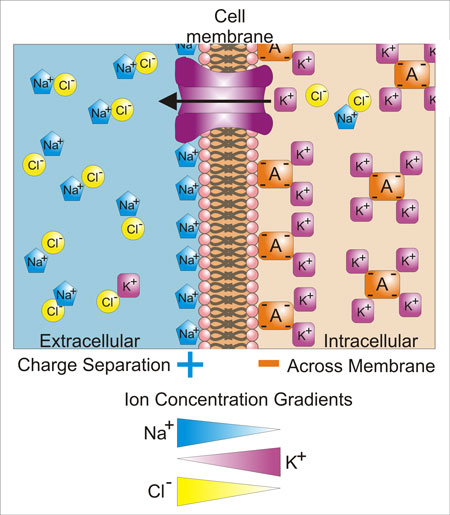 |
Different ions on opposite sides of a cellular membrane. The blue, yellow and purple ions are able to pass through the channels; the red ions are not able to permeate the cellular membrane. (Image: Wikimedia/Vojtech Dostal)
|
These pumps in human cells are controlled by a signal given by the cell itself or its environment – they may react to a change in potential along a neuron for example, and then automatically “reset” the potential once the signal has passed. However, in a number of exotic organisms (e.g. in certain marine bacteria) these pumps do not react to intracellular signals, but rather to light.
|
These unusual ion pumps could potentially be used to control nerve impulses in human cells (and in any other types of cells): by taking a neuron membrane and “implanting” two types of these pumps (to “charge” and “discharge” a cell) that react to light of different wavelengths (i.e. different colours), it will be possible to shine laser beams (different wavelengths at different times) onto individual nerve cells and therefore control the movement of ions across a neural membrane and the transmission of nerve signals through the neuron.
|
 |
Laser pointers in various different colours. The different colours of light will potentially be used to activate the proteins responsible for different ions. (Image: Wikimedia/Netweb01)
|
Optogenetics: a new field of science
|
In 2002, a team of scientists led by Prof. Dr. Ernst Bamberg and Prof. Dr. Peter Hegemann demonstrated that this method can be used to control the potential difference on opposite sides of the cellular membrane in cells of a type of green algae. A few years later it was demonstrated that the approach could be used not only on individual cells, but also on larger organisms – even mice.
|
The scientists used three types of pumps to pump the ions. The first pumps were non-specific channels (e.g. channelrhodopsins). When they are exposed to light they let certain positive ions pass through, but it is not possible to control the type of ion (e.g. potassium or sodium). The second type were various halorhodopsins, which only pump negative chlorine ions through the membrane. The third type were proton pumps that are capable of transferring protons through the cellular membrane. The problem is that the first two types are not enough to control nerve signals with a good level of accuracy and the third type of pump has a negative effect on the cell – it alters the pH in its cytoplasm, which negatively affects the function of its internal mechanisms.
|
 |
Dimensions of various ions that pass through a membrane. In a solution, the proton H+ is most often found in the form of a hydroxyl ion – a hydrated water molecule. (Image: MIPT)
|
For a smoother “discharge”, it is better to use pumps that only pump potassium or sodium ions, which is what neurons themselves normally use to do this. Using “wild-type” pumps taken directly from exotic bacteria is not always successful – they often need to be modified so they are only sensitive to a certain range of wavelengths (or to put it more simply, so that they react to a particular colour of light). Or they need to be made to allow a different type of ion to pass through. In order to be able to make these modifications, it is important to know the structure and function of these pumps.
|
The function of KR2
|
In their paper, the authors used their previous research ("Crystal structure of a light-driven sodium pump") to analyse the structure of the light-driven sodium pump KR2 in various states. They were then able to use this analysis to propose a model of the molecular function of this pump.
|
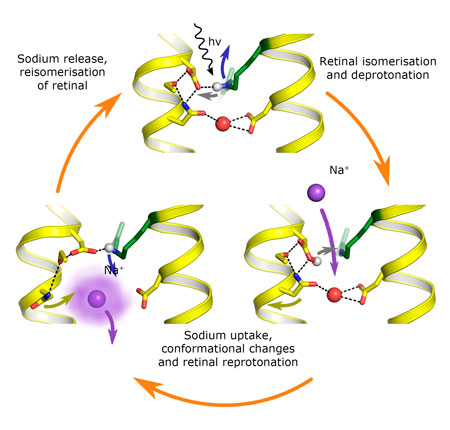 |
Function of the KR2 protein. It all starts when retinal (top of the picture) is exposed to light. The sodium ion is shown in purple. (Image courtesy of the authors of the study)
|
When light hits the retinal (the light-driven element in the structure of a pump), it undergoes an isomerization (change in molecular arrangement) and allows a sodium ion to enter the protein. When the sodium enters the protein, the retinal changes again preventing the sodium from going back in the direction it came from. When the sodium is released again, the protein returns to its original state and is ready to absorb the next photon.
|
The authors also analysed how the pump function is affected by the fact that in a membrane it often forms oligomers – groups of several proteins close to each other (the particular protein analysed forms pentamers – a complex of five proteins).
|
It was found that the width of the “gates” enabling an ion to pass through in the proteins in these complexes is slightly bigger than in monomeric KR2 – 12 angstroms compared to 10. In other pumps with known structures responsible for proton transfer, this distance can also be measured and it will be less than in KR2.
|
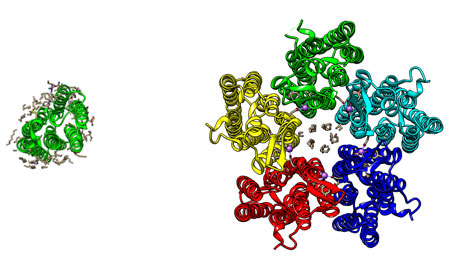 |
Monomer (left) and pentamer (right) of the protein KR2. In the pentamer, five similar molecules are joined together in one large complex, which slightly affects the structure of each molecule. (Image: MIPT)
|
"We believe that the value of approximately 12 angstroms may be the minimum value for a protein to be able to transfer a charged ion instead of a proton. Knowing this threshold value, we can use computer methods to modify other pumps in this way so they transfer the ions we need. Of course, this will require further adjustments in a number of key places in the protein,” commented Vitaly Shevchenko, an author of the paper and a researcher of MIPT’s Laboratory for Advanced Studies of Membrane Proteins.
|
Conclusion
|
The protein KR2 has the potential to become a key tool in the new science of optogenetics. Its creators were recently awarded a Breakthrough Prize (you can read about this in more detail here for example). Research in this field is also being conducted at MIPT and it was recently announced that a centre would be established for aging and age-related desiases, which is also planned to include an optogenetics laboratory headed by Professor Valentin Gordeliy – the corresponding author of the paper.
|